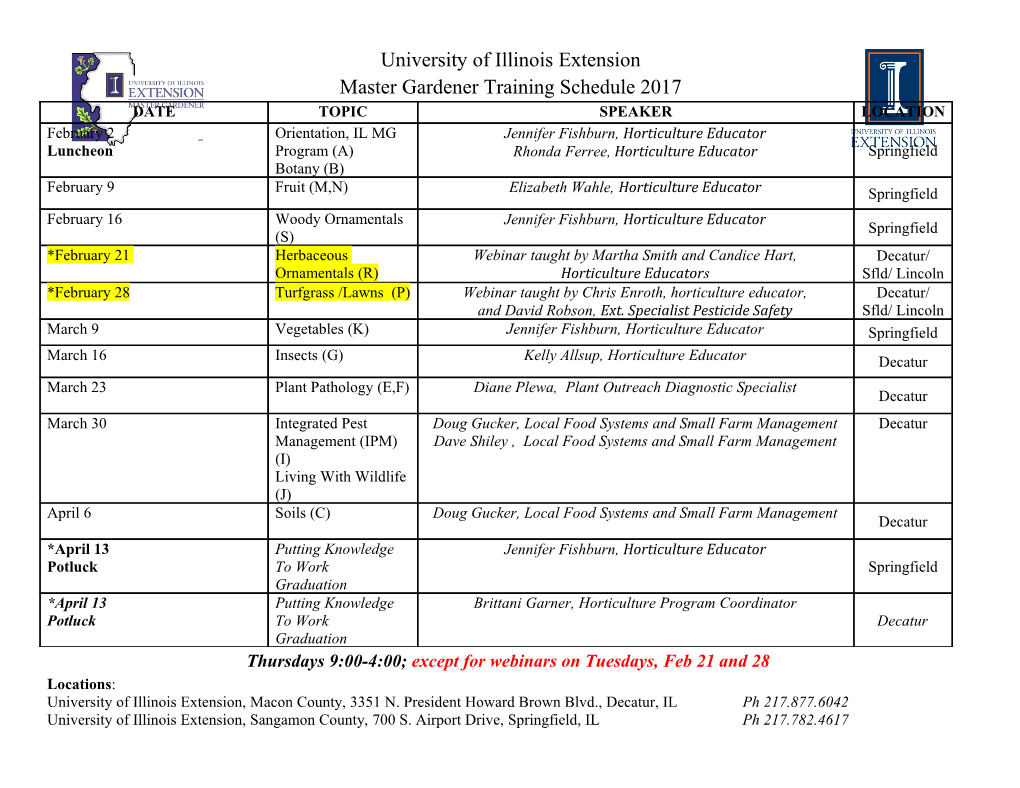
44th Lunar and Planetary Science Conference (2013) 2422.pdf THE SPATIAL AND TOPOGRAPHIC DISTRIBUTION OF PIT CRATERS ON MERCURY. Jeffrey Gillis- Davis1, Timothy A. Goudge2, James W. Head2, Zhiyong Xiao3,4, and Paul K. Byrne5.1University of Hawaii – Manoa, Hawaii Institute of Geophysics and Planetology, 1680 East-West Road, Honolulu, HI 96822, USA ([email protected]); 2Department of Geological Sciences, Box 1846, Brown University, Providence, RI 02912, USA; 3Lunar and Planetary Laboratory, University of Arizona, Tucson, AZ 85719, USA; 4China University of Geo- sciences (Wuhan), Wuhan, Hubei, P. R. China, 430074; 5Department of Terrestrial Magnetism, Carnegie Institution of Washington, Washington DC, 20015, USA. Introduction: Global images of Mercury from the Results: Fig. 1 shows the global distribution of pit MESSENGER spacecraft have revealed more than 100 craters larger than 10 km. We categorized pit craters by pit craters [1,2], rimless depressions ranging from 10 geologic setting and by shape. Pits are found in three km to almost 80 km. Although a number of pits smaller different geologic settings: The floors of impact craters than 10 km have also been identified on Mercury [3,4], (77%), within intercrater plains (17%), and within ba- we make a distinction on the basis of size because pits sins (6%) (Fig. 1). Pit craters hosted within an impact less than 10 km across can be formed by multiple crater vary in shape from irregular, to arcuate, to circu- mechanisms, and the image resolution needed to assess lar. The shape of the pit crater is influenced by the loca- detailed morphology and discern a formation mecha- tion of the pit within its host impact crater. Pits more nism is not always adequate. We do not consider such centrally located on an impact crater floor are typically smaller features further in this work. circular, whereas those off-center tend to be elongate The size range of the pits mapped here, 10–80 km, and arcuate, conforming to the rim or an interior ring of is comparable to that for large calderas on Earth [5], the host crater. It has been inferred [1] that impact frac- Venus [6,7], and Mars [8,9]. Evidence in favor of a turing facilitated the loci of magma ascent and con- shallow, non-effusive igneous origin for Mercury’s pit trolled pit crater shape. In contrast, all pits outside of craters [1,3,4,10] includes (1) lack of evident impact impact craters are classified as irregularly shaped. The ejecta; (2) lack of raised rims; (3) irregular and often absence of arcuate intercrater pits suggests that impact arcuate outlines, unlike circular or near-circular outlines fracturing influenced pit morphology within craters. of impact craters; (4) general association with bright On a regional scale, some pit craters tend to be dis- and spectrally red material interpreted to be pyroclastic tributed in clusters or groupings, whereas others are deposits, (5) spatial association with volcanic smooth more isolated. We observe that in three locations the plains; and (6) lack of observable lava flows that cut groupings appear linear – connected by a white line in their rims. Gillis-Davis et al. [1] proposed a two-step Fig 1. The linear nature of some of the clusters suggests mechanism of formation: (1) emplacement of a near- a possible connection to a near-surface dike, under a surface magma chamber beneath an impact crater floor, scenario whereby a dike propagates to the near surface and (2) withdrawal of magma from or degassing of such a reservoir, thus weak- ening the chamber's roof, leading to roof collapse and the formation of the pit cra- ter. A variation on this theme (dike emplacement, degassing, explosive erup- tion, and collapse) has also been proposed [11]. Here we study the loca- tion, geologic context, and topographic occurrence of pit craters to examine fac- tors that might control their distribution (e.g., faults, thickness and density struc- Fig. 1. Distribution of pit craters on Mercury by morphologic type. MESSEN- ture of the crust, neutral GER albedo image mosaic, simple-cylindrical projection, centered on 0º, 180º. buoyancy zone, and persis- Basin locations, diameters, and confidence (green for certain yellow for prob- tence of shallow magma able) [13]. Labeled basins include Beethoven (B), Caloris (C), Chong-Gauguin reservoirs). (C-G), Matisse-Repin (M-R), Rembrandt (R), and Vy-Asa (V-A), and probable basins b30, b54, and b64 [13]. 44th Lunar and Planetary Science Conference (2013) 2422.pdf and has sufficient exsolved gas population of pit craters toward to form pits but is still too deep lower and middle elevations. No to form a graben [12]. All three predominance of pit craters in locations are in close proximity low elevations was observed. to visible lobate scarps. Al- But if pits formed in areas of low though no timing relationship topography they may be buried between the pits and the scarps by subsequent lava. For instance, can be established, the scarps do the northern volcanic plains oc- indicate the fractured nature of cupy an expansive area of low the crust in these locations. Fur- topography that has experienced thermore, the linear grouping of extensive volcanic resurfacing pits centered on 50°S, 10°E is [18]. However, these plains were also located along the rim of a probably formed by a high- possible basin, b54 in [13]. volume eruption [18], which Pit craters also occur along favors direct ascent of magma visible and possible basin rings. over stalling of magma in the At least eight pit craters, all but crust and subsequent formation one hosted within an impact cra- Fig. 2. Comparison of pit crater eleva- of a reservoir that would later ter, occur along known basin tion (black histogram) and histogram of collapse and form a pit. rims. Four pits are on the rim of northern hemisphere elevations, relative Conclusions: The presence Caloris, and one pit occurs on to a sphere of radius 2440 km [17]. of pit craters suggests that shal- the rim of each of the following low magma reservoirs existed basins: Beethoven, Chong-Gauguin, Rembrandt, and within the crust. Eruptions were not sustained over a Vy-Asa (Fig. 1). The lone intercrater pit in this category long duration of time, as that would have led to the con- occurs on the rim of Rembrandt basin. The locations of struction of large edifices, which are not observed. On three pit craters found to the west of the Matisse-Repin the basis of the spatial and topographic controls consid- basin, though not on the mapped rim of the basin, may ered here, we find that structural features play an im- have been influenced by the structure of the basin. portant but not controlling role in pit crater formation. There are also seven additional pit craters that occur on Approximately half of cataloged pits are associated the rims of probable basins: Five are on the rim of basin with observed or probable basin rims or form linear b30 (diameter 1390 km; 15.9°N, 21°E) [13], and one trends, which we infer are associated with shallow each is on the rim of Derzhavin-Sor Juana (diameter dikes. The remaining pit craters are more randomly but 580 km; 52.3°N, 28.3°E [13]) and b64 (diameter 310 not evenly distributed. The topographic distribution of km; 16.2°S, 160.8°E [13]). These pits may be calderas the remaining pit craters corresponds with the distribu- caused by intrusive dikes along subsurface fractures tion of topographic elevations in the northern hemi- that were created during basin formation. sphere, which suggests either a coincidental neutral Nonetheless, about half of the pit craters occur buoyancy zone in the mid level of the crust or, more without an apparent association to a structural (e.g., likely, that outside of structural controls pit crater for- basin rim or dike) feature. Many of these enigmatic pits mation is a process that is not influenced by the eleva- occur in an area of high topography south of Caloris tion at which it operates. (~10°S, 150°E), and some are scattered throughout the References: [1] Gillis-Davis, J.J., et al. (2009) EPSL, 285, 243. northern half of the western hemisphere. Furthermore, [2] Gillis-Davis, J.J., et al. (2012) LPS, 43, 2288. [3] Goudge, T.A., et pits do not occur along the margins of major volcanic al. (2012) LPS, 43, 1325 [4] Kerber, L., et al. (2011) Planet. Space deposits in the manner of floor-fractured craters and Sci., 59, 1895. [5] Lipman, P.W. (1999) in Encyclopedia of Volca- noes, p. 643. [6] Parfitt, L., and L. Wilson (2008) in Fundamentals of mare deposits on the Moon [14,15]. Physical Volcanology, p. 190. [7] Crumpler, L.S., et al. (1996) in In comparing the topographic elevations of pit cra- Venus II, p. 697. [8] Crumpler, L.S., et al. (1996) J. Geol. Soc. Lon- ters measured from global topography [16] with the don, 110, p.307. [9] Mouginis-Mark, P.J., et al. (2007), in The Geol- hypsometric curve derived for the northern hemisphere ogy of Mars: Evidence from Earth-Based Analogs, p. 71. [10] Head, from MESSENGER laser altimeter data [17], the two J.W., et al. (2008) Science, 321, 69. [11] Jozwiak, L.M., and J.W. distributions are similar (Fig. 2). The hypsometric dis- Head, (2012) LPSC 43, 2424. [12] Head, J.W., and L. Wilson (1992) GCA, 56, 2155. [13] Fassett, C.I., et al. (2012) JGR, 117, E00L08, tribution of pits may simply reflect the likelihood that doi:10.1029/2012JE004154. [14] Schultz, P.H., Moon, 15, 241. [15] the greater the areal extent of a given elevation repre- Jozwiak L.M., et al.
Details
-
File Typepdf
-
Upload Time-
-
Content LanguagesEnglish
-
Upload UserAnonymous/Not logged-in
-
File Pages2 Page
-
File Size-