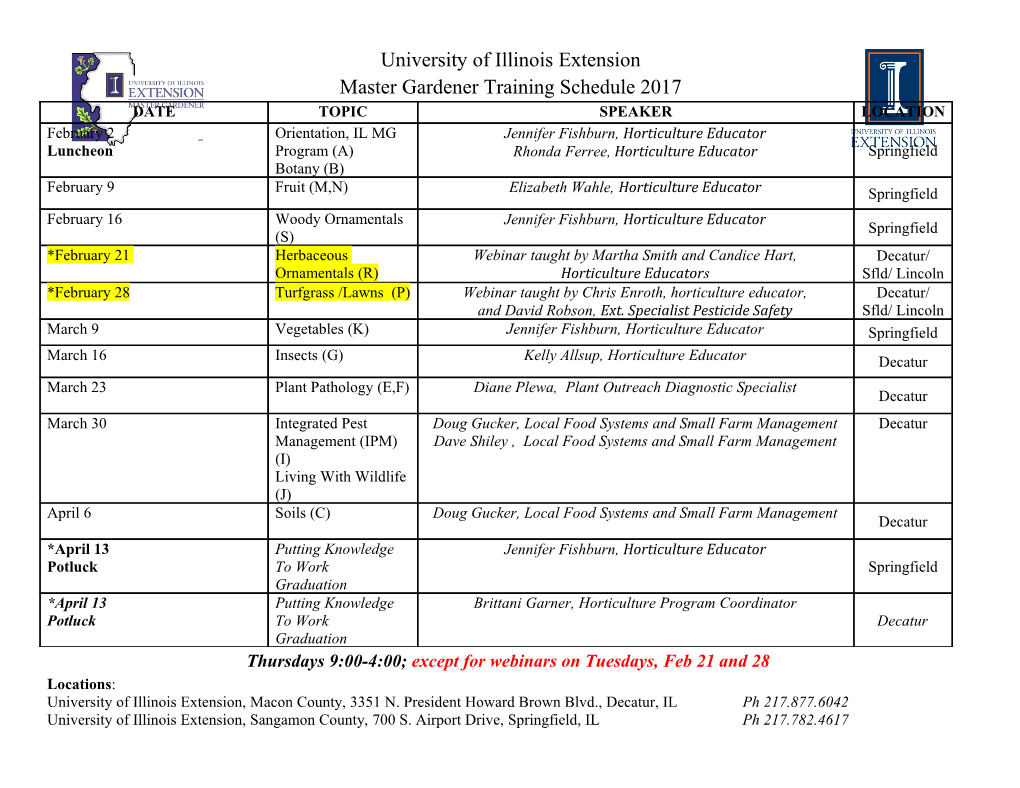
bioRxiv preprint doi: https://doi.org/10.1101/765578; this version posted April 27, 2020. The copyright holder for this preprint (which was not certified by peer review) is the author/funder, who has granted bioRxiv a license to display the preprint in perpetuity. It is made available under aCC-BY-NC-ND 4.0 International license. 1 2 3 Spatial and phylogenetic structure of Alpine stonefly community assemblages across 4 seven habitats using DNA-species 5 6 Maribet Gamboa1, Joeselle Serrana1, Yasuhiro Takemon2, Michael T. Monaghan3,4, Kozo 7 Watanabe1,5 8 9 10 1Ehime University, Department of Civil and Environmental Engineering, Matsuyama, Japan 11 12 2Water Resources Research Center, Disaster Prevention Research Institute, Kyoto University, 13 6110011 Gokasho, Uji, Japan 14 15 3Leibniz-Institute of Freshwater Ecology and Inland Fisheries (IGB), Müggelseedamm 301, 16 12587 Berlin, Germany 17 18 4Institut für Biologie, Freie Universität Berlin, Königin-Luise-Str. 1-3, 14195 Berlin, Germany 19 20 5Ehime University, Center Marine Environmental Studies (CMES), Matsuyama, Japan 21 22 23 24 25 26 bioRxiv preprint doi: https://doi.org/10.1101/765578; this version posted April 27, 2020. The copyright holder for this preprint (which was not certified by peer review) is the author/funder, who has granted bioRxiv a license to display the preprint in perpetuity. It is made available under aCC-BY-NC-ND 4.0 International license. 27 Abstract 28 1. Stream ecosystems are spatially heterogeneous, with many different habitat patches 29 distributed within a small area. The influence of this heterogeneity on the biodiversity of 30 benthic insect communities is well documented; however, studies of the role of habitat 31 heterogeneity in species coexistence and community assembly remain limited. Here, we 32 asked how habitat heterogeneity influences spatial structure (beta biodiversity) and 33 phylogenetic structure (evolutionary processes) of benthic stonefly (Plecoptera, Insecta) 34 communities. 35 2. We sampled 20 sites along two Alpine rivers, including seven habitats in four different 36 reaches (headwaters, meandering, bar-braided floodplain, and lowland spring-fed). We 37 identified 21 morphological species and delineated 52 DNA-species based on sequences 38 from mitochondrial cox1 and nuclear ITS markers. Using DNA-species, we first analysed 39 the patterns of variation in richness, diversity, and composition by quantifing the 40 contribution of each of the four reaches and seven habitats to the overall DNA-species 41 diversity using an additive partition of species diversity analysis and distance-based 42 redundancy analysis. Using gene-tree phylogenies, we assessed whether environmental 43 filtering led to the co-occurrence of DNA-species using a two-step analysis to find a 44 phylogenetic signal. 45 3. The four reaches significantly contributed to DNA-species richness; with the meandering 46 section displaying the highest contribution. However, we found that habitats had an effect 47 on DNA-species diversity, where glide, riffle, and pool influenced the spatial structure of 48 stonefly communities possibly due to a high species turnover. 49 4. Among the habitats, the pool showed significant phylogenetic clustering, suggesting high 50 levels of evolutionary adaptation and strong habitat filtering. This community structure may 51 be caused by long-term stability of the habitat and the similar requirements for co-ocurring 52 species. 53 5. Our study shows the importance of different habitats on the spatial and phylogenetic 54 structure of stonefly community assemblies and sheds light on the habitat-specific diversity 55 that may help improve conservation practices. 56 57 58 KEYWORDS 59 DNA-species, habitats, spatial structure, phylogenetic structure, Plecoptera 60 bioRxiv preprint doi: https://doi.org/10.1101/765578; this version posted April 27, 2020. The copyright holder for this preprint (which was not certified by peer review) is the author/funder, who has granted bioRxiv a license to display the preprint in perpetuity. It is made available under aCC-BY-NC-ND 4.0 International license. 61 1. INTRODUCTION 62 63 Understanding species diversity patterns and processes governing species coexistence in a 64 community is one of the fundamental research objectives in ecology and biodiversity. The 65 development of effective and suitable biodiversity conservation strategies requires knowledge 66 of the processes driving community variation (Socolar, Gilroy, Kunin, & Edwards, 2016) along 67 temporal and spatial gradients (Anderson et al., 2011). Habitat specialisation is known to 68 influence changes in community composition along temporal and spatial gradients (e.g., Wiens 69 et al., 2010); however, this change depends on the degree of specialisation of the species 70 within a community (Rádková et al. 2014). 71 Stream ecosystems provide one of the most heterogeneous landscapes, given the 72 dynamic interaction between spatial elements (e.g., topography) and ecological process (e.g., 73 hydrology) (Benda et al., 2004; Tockner & Stanford, 2002). This interaction creates a variety of 74 different habitats throughout the longitudinal (upstream-downstream) gradient of the river, 75 classified as lotic (running water) and lentic (standing water) (Dobson & Frid, 1998; Calow & 76 Petts, 1996; Hauer & Lamberti, 1996). At the biogeographic scale, habitat heterogeneity in a 77 river channel defines environmental patches that impact aquatic community composition and 78 species distribution (Brasil, Da Silva, Batista, Olivera, & Ramos, 2017; Dias-Silva, Cabetter, 79 Juen, & De Marco, 2013); while at the local scale, creates environmental filtering to sort species 80 with similar requirements (Saito, Cianciaruso, Siqueira, Fonseca-Gessner, & Povoine, 2016; 81 Mykra, Heino, &, Muotka, 2007; Webb, Ackerly, McPeek, & Donoghue, 2002). Aquatic insects 82 are abundant, diverse, and broadly distributed (Lancaster & Downes, 2013). Many studies have 83 reported a strong positive relationship between aquatic insects species diversity and spatial 84 habitat heterogeneity (e.g. Astorga et al., 2014; Karaus, Larsen, Guillong, & Tockner, 2013; 85 Arscott, Tockner, & Ward, 2005; Benda et al., 2004; Batista, Buss, Dorville, & Nessimian, 2001), 86 however, these were only based on one spatial scale, where the relationship between assembly 87 process on both biogeographical and local scales remains limited to a few studies. Thus, 88 understanding local trends in biodiversity is crucial to describe the interaction between habitat 89 and species diversity at different scales. 90 One of the challenges in biodiversity research is that of species delimitation. Due to 91 limitations in taxonomic expertise and incomplete descriptions of taxonomic groups, many 92 species may remain unidentified or unclassified (Bickford et al., 2007), making it difficult to 93 describe local biodiversity. Genetic methods developed over the last decade have helped with 94 species identifications and the clarification of species boundaries of a wide range of taxa, 95 including aquatic insects (e.g., Gamboa & Arrivillaga-Henriquez, 2019; Serrana, Miyake, 96 Gamboa, & Watanabe, 2019; Gattoliatt, Rutschmann, Monaghan, & Sartori, 2018). The 97 Generalized Mixed Yule Coalescent model (GMYC; Pons et al., 2006) is a method commonly 98 used to inditify putative DNA-species, by quantifying the transition between inter- and intra- 99 species branching events on an ultrametric tree (i.e., where the distance from the root of every 100 branch is equal), using molecular markers, such as mitochondrial DNA (Fujisawa & bioRxiv preprint doi: https://doi.org/10.1101/765578; this version posted April 27, 2020. The copyright holder for this preprint (which was not certified by peer review) is the author/funder, who has granted bioRxiv a license to display the preprint in perpetuity. It is made available under aCC-BY-NC-ND 4.0 International license. 101 Barraclough, 2013; Mynott, Webb, & Suter, 2011), or based on the congruency between 102 mitochondrial and nuclear DNA (Rutschmann et al., 2016). 103 Stream ecologists have reported that local environmental heterogeneity may be the 104 strongest driver of community assembly patterns using putative DNA-species diversity of 105 aquatic insects along the river (Astorga et al., 2014; Baselga et al., 2013; Finn, Bonada, Múrria, 106 & Hughes, 2011). However, the majority of these observations is based on DNA-species 107 diversity changes along the river’s longitudinal gradient (Finn, Zamora-Muñoz, Múrria, Sáinz- 108 Bariáin, & Alba-Tercedor, 2013; Gill, Harrington, Kondratieff, Zamudio, Poff, & Funk, 2013; 109 Jackson, Battle, White, Pilgrim, Stein, Miller, & Sweeney, 2013; Finn et al., 2011; Finn & LeRoy 110 Poff, 2011; Hughes, Schmidt, & Finn, 2009), where the influence of other river dimensions (e.g., 111 habitats), has only been described in few species, such as beetles (Ribera & Vogler, 2008) or 112 caddisflies (Marten, Brandle, & Brandl, 2006). Therefore, investigating variations in spatial 113 patterns of aquatic insects community composition using DNA-species in the river channel and 114 their relationship with habitat heterogeneity will help to improve the understanding of the 115 process of community assembly in aquatic ecosystems. 116 The understanding of the community assembly not only has focused on spatial patterns 117 and composition changes analysis. The phylogenetic signal has been proposed as an 118 additional insight to understand the drivers of community
Details
-
File Typepdf
-
Upload Time-
-
Content LanguagesEnglish
-
Upload UserAnonymous/Not logged-in
-
File Pages30 Page
-
File Size-