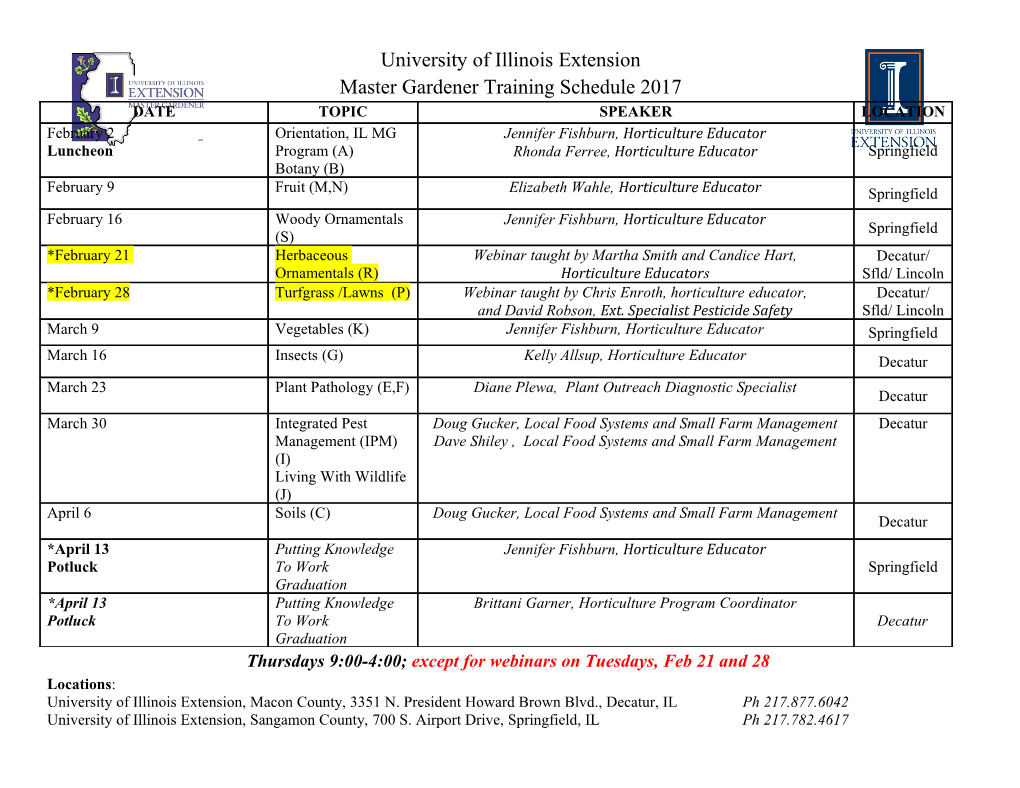
Space Sci Rev DOI 10.1007/s11214-012-9894-0 High-Energy Atmospheric Physics: Terrestrial Gamma-Ray Flashes and Related Phenomena Joseph R. Dwyer · David M. Smith · Steven A. Cummer Received: 1 December 2011 / Accepted: 4 May 2012 © The Author(s) 2012. This article is published with open access at Springerlink.com Abstract It is now well established that both thunderclouds and lightning routinely emit x-rays and gamma-rays. These emissions appear over wide timescales, ranging from sub- microsecond bursts of x-rays associated with lightning leaders, to sub-millisecond bursts of gamma-rays seen in space called terrestrial gamma-ray flashes, to minute long glows from thunderclouds seen on the ground and in or near the cloud by aircraft and balloons. In particular, terrestrial gamma-ray flashes (TGFs), which are thought to be emitted by thun- derclouds, are so bright that they sometimes saturate detectors on spacecraft hundreds of kilometers away. These TGFs also generate energetic secondary electrons and positrons that are detected by spacecraft in the inner magnetosphere. It is generally believed that these x-ray and gamma-ray emissions are generated, via bremsstrahlung, by energetic runaway electrons that are accelerated by electric fields in the atmosphere. In this paper, we review this newly emerging field of High-Energy Atmospheric Physics, including the production of runaway electrons, the production and propagation of energetic radiation, and the effects of both on atmospheric electrodynamics. Keywords Thunderstorms · Lightning · x-rays · Gamma-rays · Energetic radiation · Atmospheric electricity J.R. Dwyer () Department of Physics and Space Sciences, Florida Institute of Technology, Melbourne, FL 32901, USA e-mail: jdwyer@fit.edu D.M. Smith Physics Department and Santa Cruz Institute for Particle Physics, University of California, Santa Cruz, CA 95064, USA e-mail: [email protected] S.A. Cummer Electrical and Computer Engineering Department, Duke University, Durham, NC 27708, USA e-mail: [email protected] J.R. Dwyer et al. 1 Introduction Despite the ubiquity of thunderstorms, lightning, and related electrical phenomena, many important electromagnetic processes in our atmosphere are poorly understood. For exam- ple, many questions remain about thundercloud electrification and discharge mechanisms, lightning initiation, propagation and attachment processes, compact intra-cloud discharges, the global electrical circuit, and transient luminous events (Rakov and Uman 2003). Tra- ditionally, these topics have been studied using classical electromagnetism. However, in the last few years, a growing body of literature has emerged that describes the production, transport and interactions of energetic particles in our atmosphere. Specifically, it is now well established that thunderclouds, lightning, and long laboratory sparks in air all produce energetic runaway electrons and accompanying x-ray and gamma-ray emissions. Terrestrial gamma-ray flashes (TGFs), bright bursts of multi-MeV gamma-rays that are seen hundreds of kilometers away by spacecraft, are particularly impressive examples of runaway electron production in our atmosphere. Moreover, such high-energy particles interact with air atoms, forming low-energy electron and ion populations that may greatly increase the conductivity of air, potentially affecting the physics of thunderclouds and lightning. We shall refer to the rapidly expanding field of energetic particle and radiation physics in terrestrial and plane- tary atmospheres, and their effects, as High-Energy Atmospheric Physics. Not only does this field impact traditional atmospheric electricity and lightning physics, it also has implications for the study of cosmic-ray extensive air showers, discharge physics, space physics, plasma physics, and aviation safety. In this paper, we shall review runaway electron production mechanisms, including rel- ativistic runaway electron avalanches, thermal runaway electron production and the rela- tivistic feedback mechanism, bremsstrahlung emissions, and x-ray and gamma-ray propa- gation. We shall also review energetic radiation observations, including terrestrial gamma- ray flashes (TGFs) and terrestrial electron beams (TEBs), gamma-ray glows from thunder- clouds, neutron observations, x-rays from lightning and laboratory sparks, and radio fre- quency emissions and observations. Finally, we shall discuss recent modeling work and implications for atmospheric phenomena such as sprites and other transient luminous events (TLEs), compact intra-cloud discharges (CIDs), thunderstorm electrification and lightning initiation, and radiation doses to individuals in aircraft. 2 High-Energy Atmospheric Physics Theory 2.1 Wilson Runaway Electrons In 1925, C.T.R. Wilson discovered the runaway electron mechanism in which fast elec- trons may obtain large energies from static electric fields in air (Wilson 1925). Specifically, when the rate of energy gain from an electric field exceeds the rate of energy loss from interactions with air then the energy of an electron will increase and it will “run away.” Run- away electrons are produced in electric fields greater than the so-called break-even field, 5 Eb = 2.18 × 10 V/m × n, corresponding to the rate that minimum ionizing electrons lose energy, where n is the density of air with respect to that at sea level. Simulations have shown that due to elastic scattering, the field required for runaway electrons to propagate large dis- tances is actually about 30 % higher than Eb (Dwyer 2003). This field is about a factor of ten below the conventional breakdown field and is comparable to maximum fields seen inside thunderclouds (Rakov and Uman 2003). Indeed, Marshall et al. (1995) found that High-Energy Atmospheric Physics: Terrestrial Gamma-Ray Flashes Fig. 1 The effective frictional force experienced by a free electron (or positron) moving through air at STP as a function of kinetic energy. The solid curve is due to inelastic scattering of the electron by air molecules, and the dashed curve indicates the effects of bremsstrahlung emission. The horizontal line shows the electric force from a 5.0 × 106 V/m electric field. Runaway electrons occur for kinetic energies greater than the threshold energy, ε>εth. In the figure, Ec is the critical electric field strength for which low-energy thermal electrons will run away, and Eb is the so-called break-even field balloon soundings inside thunderclouds often measured maximum electric fields near the break-even field, suggesting a possible connection between lightning initiation and runaway election production (also see Stolzenburg et al. 2007). Figure 1 shows the rate of energy loss of an energetic electron moving in air (effective frictional force). The plot also shows that rate of energy gain from a strong electric field (horizontal line). As can be seen, in order for an electron to run away, it must have an initial kinetic energy above the threshold, εth. Such energetic “seed” electron, with energies above εth, may be provided from an external source such as cosmic-rays or radioactive decays. Note that the kinetic energy, εth, required for the seed particles decreases rapidly with increasing electric field. When the electric field is increased above the critical field, Ec, above the energy loss curve for all kinetic energies, then all free electrons may run away, and, in particular, the thermal population created at low energies may run away. This mechanism is usually called “cold runaway” or “thermal runaway,” and does not require any external seed particles (Gurevich 1961). According to Wilson’s original work, the energetic seeds that result in runaway electrons are all provided by external sources such as cosmic-rays (or radioactive decays). Therefore, for each atmospheric cosmic-ray secondary electron that arrives, at most one runaway elec- tron may be generated. More concisely, each atmospheric cosmic-ray secondary electron may become a runaway electron in a sufficiently large electric field. Although a runaway electron may gain energy and travel farther than the seed particle without an electric field, this mechanism will not produce large fluxes of runaway electrons (McCarthy and Parks 1992), especially the large fluxes known to be associated with terrestrial gamma-ray flashes (TGFs). On the other hand, it is possible for thermal runaway to provide the energetic seeds, which subsequently experience additional energy gain and avalanche multiplication. This combination could potentially explain TGFs, as will be discussed below. J.R. Dwyer et al. 2.2 Relativistic Runaway Electron Avalanches (RREAs) 2.2.1 Introduction In 1992, Gurevich, Milikh and Roussel-Dupré showed that when Møller scattering (electron- electron elastic scattering) is included, the runaway electrons described by Wilson will un- dergo avalanche multiplication, resulting in a large number of relativistic runaway electrons for each energetic seed electron injected into the high-field region (Gurevich et al. 1992; Gurevich and Zybin 2001). This avalanche mechanism is commonly referred to as the Rel- ativistic Runaway Electron Avalanche (RREA) mechanism (Babich et al. 1998, 2001a). Al- though Wilson appears to have been aware of the runaway electron avalanche multiplication (Williams 2010), referring to it as a “snowball effect,” he did not provide quantitative calcu- lations of the avalanche properties. The runaway electron avalanche threshold electric field was estimated by Symbalisty et al. (1998) to be in the range 2.83–3.05 × 105 V/m × n, where n is the density of air
Details
-
File Typepdf
-
Upload Time-
-
Content LanguagesEnglish
-
Upload UserAnonymous/Not logged-in
-
File Pages64 Page
-
File Size-