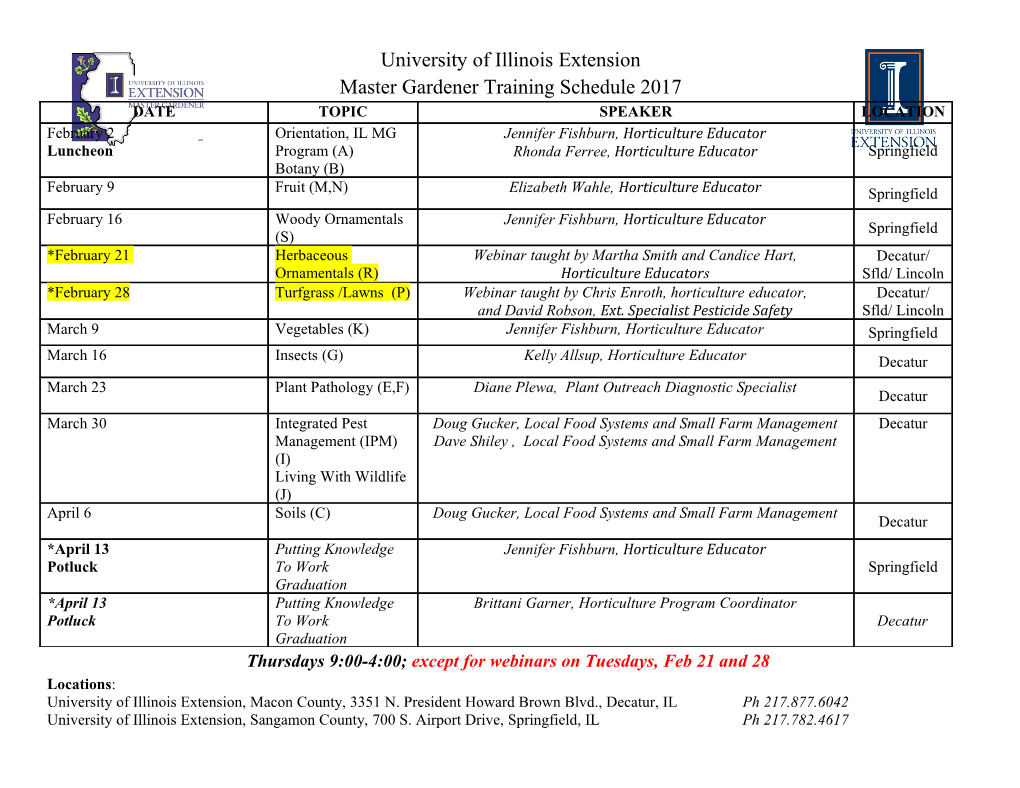
SHEAR THICKENING IN COLLOIDAL SILICA CHEMICAL MECHANICAL POLISHING SLURRIES by Anastasia Krasovsky A thesis submitted to the Faculty and the Board of Trustees of the Colorado School of Mines in partial fulfillment of the requirements for the degree of Master of Science (Chemical Engineering). Golden, Colorado Date _________________________ Signed: _________________________ Anastasia Krasovsky Signed: _________________________ Dr. Matthew W. Liberatore Thesis Advisor Golden, Colorado Date _________________________ Signed: _________________________ Dr. David W. M. Marr Professor and Head Department of Chemical and Biological Engineering ii ABSTRACT Chemical mechanical polishing (CMP) is used by the semiconductor manufacturing industry to polish materials under high shear for the fabrication of microelectronic devices such as computer chips. Under these high shear conditions, slurry particles can form agglomerates causing defects, which cost the semiconductor industry billions of dollars annually. The shear thickening behavior of colloidal silica CMP slurries (28-38 wt%) under high shear was studied using a rotating rheometer with parallel-plate geometry. The colloidal silica slurries showed continuous thickening and irreversible behavior at high shear rates (>10,000 s-1). Changing the silica concentration, adding monovalent chloride salts (NaCl, KCl, CsCl, and LiCl), adjusting the pH (pH 4 to pH 10.5), and mixing two particle sizes (d = 20 nm and 100 nm) within the slurry altered the thickening behavior of the slurries. Shear thickening behavior can be eliminated with certain large to small particle ratios. iii TABLE OF CONTENTS ABSTRACT…………………………………………………………………………………….. iii LIST OF FIGURES……………………………………………………………………………... vi LIST OF TABLES…………………………………………………………………………...... viii LIST OF SYMBOLS……………………………………………………………………………. ix LIST OF ABBREVIATIONS……………………………………………………………………. x CHAPTER 1 BACKGROUND………………………………………………………………… 1 1.1 Chemical Mechanical Polishing…………………………………………………. 1 1.2 High Shear Rheology…………………………………………………………….. 5 1.2.1 Common Techniques and Geometries…………………………………… 5 1.2.2 Parallel-Plate High Shear Rheology……………………………………... 6 1.3 Chemical Mechanical Polishing Slurries………………………………………… 8 1.4 Stability of Colloidal Silica……………………………………………………... 10 CHAPTER 2 SHEAR THICKENING OF CHEMICAL MECHANICAL POLISHING SLURRIES UNDER HIGH SHEAR WITH ADDED ELECTROLYTE……… 14 2.1 Abstract…………………………………………………………………………. 14 2.2 Introduction……………………………………………………………………... 14 2.3 Experimental Methods………………………………………………………...... 16 2.4 Effect of Electrolyte on Shear Thickening…………………………………….... 17 2.5 Varying Silica Concentration Under Constant Salt Concentration……………... 22 2.6 Conclusions……………………………………………………………………... 24 CHAPTER 3 PARTICLE SIZE AND pH IMPACT ON SHEAR THICKENING OF COLLOIDAL SILICA CMP SLURRIES……………………………………… 26 iv 3.1 Abstract…………………………………………………………………………. 26 3.2 Introduction……………………………………………………………………... 26 3.3 Experimental Methods………………………………………………………….. 28 3.4 Shear Thickening Behavior Examined in a Range of pH Values………………. 29 3.5 Mixtures of Small and Large Colloidal Silica Particle Slurries………………… 33 3.6 Conclusions……………………………………………………………………... 36 CHAPTER 4 CONCLUSIONS AND RECOMMENDATIONS……………………………... 38 4.1 Conclusions……………………………………………………………………... 38 4.2 Recommendations………………………………………………………………. 39 REFERENCES CITED…………………………………………………………………………. 40 v LIST OF FIGURES Figure 1.1 Schematic of the CMP process, where Ωp and Ωc are the rotational speeds of the platen and wafer carrier, respectively. Adapted from Gokale and Moudgil [1]………………………………………………………………………. 2 Figure 1.2 Top (a), angled (b), and side view (c) of a concentric ring patterned polyurethane polishing pad used for experimentation…………………………… 2 Figure 1.3 Cross-section schematic of the damascene processing method for the sequential deposition of the: (a) dielectric, (b) diffusion barrier, and (c) interconnecting Cu layers. (d) Extra Cu must be removed via CMP in order to create a planarized surface for depositing the next layer of interconnects. Adapted from Matijevic and Babu [2].…………………………… 4 Figure 1.4 Schematic of a parallel-plate rheometer…………………………………………. 7 Figure 1.5 Transmission electron microscope (TEM) image of colloidal silica…………….. 9 Figure 1.6 Total interaction energy as predicted by DLVO theory versus particle separation distance between two hard spheres………………………………….. 11 Figure 2.1 Steady shear rate ramp (filled symbols) and reduction (open symbols) for 30wt% silica slurry with an added electrolyte concentration of 0.17 M KCl (black circles), 0.17 M NaCl (blue diamonds), 0.17 M CsCl (green triangles), 0.17 M LiCl (red squares), and no salt added (orange diamonds)……………… 18 Figure 2.2 Viscosity of small silica particles with a weight percent of 30% with an added electrolyte concentration of 0.17 M during shear rate ramp and reduction………………………………………………………………………... 19 Figure 2.3 Schematic of the adsorption of Cs+, K+, Na+, and Li+ onto a hydrated silica surface. Small and more hydrated counterions (Na+ and Li+) penetrate deeper into the hydration layer of silica than larger, weakly hydrated cations (Cs+ and K+). This figure is an adaption of a graphic published in Colic et al. [3]…………………………………………………………………... 22 Figure 2.4 Steady shear rate ramp (filled symbols) and reduction (open symbols) for a 28 wt% (green diamonds), 30 wt% (orange circles), 33 wt% (red diamonds), 36 wt% (black squares), and 38 wt% (blue triangles) silica slurry with an NaCl concentration of 0.17 M………………………………………………….. 23 vi Figure 2.5 Viscosity of small silica particles at 28 wt%, 30 wt%, 33 wt%, 36 wt%, and 38 wt% with an added sodium chloride concentration of 0.17 M during shear rate ramp and reduction…………………………………………………... 23 Figure 3.1 Steady shear rate ramp (filled symbols) and reduction (open symbols) for a 33 wt% small particle silica slurry pH adjusted with NaOH or HCl to pH 4 (black squares), pH 7 (blue diamonds), pH 9 (orange triangles), pH 10 (green circles), and pH 10.5 (red triangles)…………………………………….. 29 Figure 3.2 Steady shear rate ramp (filled symbols) and reduction (open symbols) for a 33 wt% large particle silica slurry pH adjusted with NaOH or HCl to pH 4 (black squares), pH 7 (blue diamonds), pH 8 (orange triangles), pH 10 (green circles), and pH 10.5 (red triangles)…………………………………….. 30 Figure 3.3 Viscosity of small silica particles (33 wt%) pH adjusted with NaOH or HCl to pH values of 4, 7, 9, 10, and 10.5 during shear rate ramp and reduction……. 30 Figure 3.4 Viscosity of large silica particles (33 wt%) pH adjusted with NaOH or HCl to pH values of 4, 7, 8, 10, and 10.5 during shear rate ramp and reduction……. 31 Figure 3.5 Steady shear rate ramp (filled symbols) and reduction (open symbols) for mixtures of small (d = 20 nm) and large (d = 100 nm) silica particles at a constant overall volume percent (23%) and a pH value of 9…………………… 33 Figure 3.6 Viscosity of mixtures of small (d = 20 nm) and large (d = 100 nm) silica particles during shear rate ramp and reduction at a constant volume percent (23%) and a pH value of 9……………………………………………………… 34 Figure 3.7 Schematic representation of how small particle spheres fit within the spaces between large particle spheres (a) and a TEM image of a mixture or small and large particle spheres (b)………………………………………….. 36 vii LIST OF TABLES Table 1.1 Applied shear rates during CMP slurry processing [4]…………………………... 1 Table 2.1 Critical shear rate for small silica particle slurries with a weight percent of 30% and an added electrolyte concentration of 0.17 M……………………... 21 Table 3.1 Conductivity and zeta potential values for small and large particles at 33 wt% for a range of pH values……………………………………………….. 32 Table 3.2 Conductivity and zeta potential values for mixtures of ratios to small and large particles at a constant volume percent (23%) and a pH value of 9……….. 35 viii LIST OF SYMBOLS Density………………………………………………………………………………………….... ρ Measured torque……………………………………………………………………………….... M Particle radius…………………………………………………………………………………...... a Rheometer gap height…………………………………………………………………………… H Rheometer plate radius…………………………………………………………………………... R Rotational speed…………………………………………………………………………………. Ω Salt concentration………………………………………………………………………………... C Shear rate………………………………………………………………………………………… � Shear stress……………………………………………………………………………………….. τ Surface tension…………………………………………………………………………………... Γ Viscosity…………………………………………………………………………………………. η ix LIST OF ABBREVIATIONS Chemical Mechanical Polishing……………………………………………………………... CMP Cabot Microelectronics Corporation………………………………………………………… CMC Derjaguin-Landau-Verwey-Overbeek theory……………………………………………… DLVO Dynamic Light Scattering…………………………………………………………………..... DLS Electrical Double Layer……………………………………………………………………… EDL Transmission Electron Microscopy………………………………………………………….. TEM x CHAPTER 1 BACKGROUND The following techniques and materials were used to study the high shear rheology of chemical mechanical polishing slurries and to examine changes to the slurry structure under shear. This chapter provides background information on chemical mechanical polishing (CMP), high shear rheology, CMP slurries, and colloidal silica stability. 1.1 Chemical Mechanical Polishing Chemical mechanical polishing (CMP) is used in the semiconductor industry to ensure that the thickness of the dielectric materials is uniform and that interconnects between the multilayer chips are reliable [5]. In the semiconductor processing industry, CMP has become the primary technique for the planarization of integrated circuits
Details
-
File Typepdf
-
Upload Time-
-
Content LanguagesEnglish
-
Upload UserAnonymous/Not logged-in
-
File Pages55 Page
-
File Size-