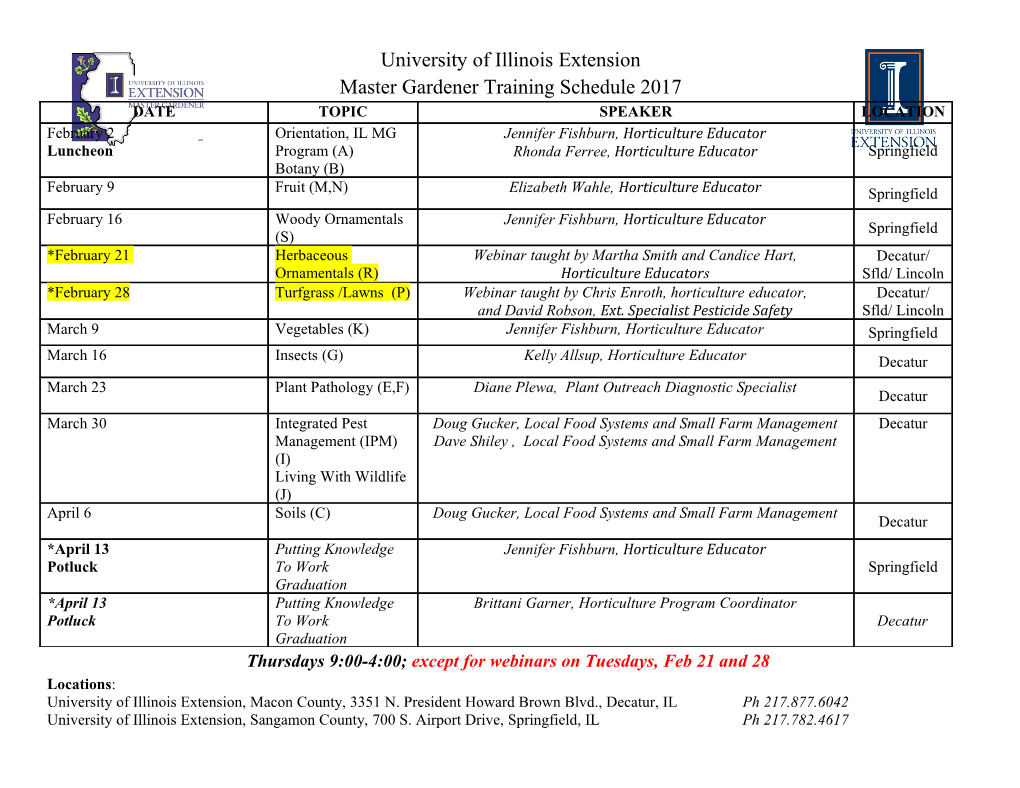
The Function of Inosine-5’-Monophosphate Dehydrogenase’s Bateman Domain in Escherichia coli Masters Thesis Presented to The Faculty of the Graduate School of Arts and Sciences Brandeis University Department of Biochemistry Lizbeth Hedstrom, Advisor In Partial Fulfillment Of the Requirements for the Degree Master of Science in Biochemistry by Sabrina Gay McDonnell May 2018 Copyright by Sabrina Gay McDonnell © 2018 Acknowledgements I am grateful for the support and guidance I have received from Prof. Lizbeth Hedstrom. She allowed me to join her lab at the beginning of my sophomore year and has continuously pushed me to think more critically about my project and better understand the work we have completed. Daniel Kats and Dr. Devi Gollapalli were my mentors when I started working in the Hedstrom lab. Together they taught me all of the fundamental techniques I needed to conduct research. When I struggled with a specific aspect of my research they helped talk through the problem and constantly pushed me to accomplish my goals. Everyone in the Hedstrom Lab supported me by giving feedback after group presentations and helped me whenever I had questions. I would especially like to thank Minjia Zhang, Ann Lawson, and Shibin Chacko. Thank you also to the Lovett Lab, specifically Vincent Sutera for assisting me in the creation of new E. coli strains and permitting me to use the Lovett Lab E. coli strain library. Thank you to the Division of Science Summer Undergraduate Research Fellowship and the M.R. Bauer Fellows program for funding my summer research endeavors and providing me a platform to share my work with the scientific community at Brandeis University. Lastly, I would like to thank my family and friends for assisting and supporting me through my three years of undergraduate research. Specifically, I’d like to thank Miriam Hood, Lena El-Teha, Ashley Klein, Jourdan Paige, Terry McDonnell, Matt McDonnell and Matthew McDonnell. Molecular graphics and analyses were performed with the UCSF Chimera package from the Resource for Biocomputing, Visualization, and Informatics at the University of California, San Francisco (supported by NIGMS P41-GM103311). iii Abstract The Function of Inosine-5’-Monophosphate Dehydrogenase’s Bateman Domain in Escherichia coli A thesis presented to the Department of Biochemistry Graduate School of Arts and Sciences Brandeis University Waltham, Massachusetts By Sabrina Gay McDonnell Inosine 5’-monophosphate dehydrogenase (IMPDH) catalyzes the rate-limiting conversion of inosine 5’-monophosphate (IMP) to xanthosine 5’-monophosphate (XMP) in the de novo purine biosynthesis pathway. Overexpression of the protein is linked to the proliferation of diseased cells, and inhibitors of IMPDH have been used as anticancer, antiviral, and immunosuppressive treatments. IMPDH is a tetramer and each monomer has two domains: a catalytic domain and a Bateman domain. The function of the Bateman domain is currently unknown. The enzyme remains catalytically active when the Bateman domain is replaced with a short peptide scar (ΔCBS). Mutations in the Bateman domain of human IMPDH 1 have been linked to hereditary diseases including retinitis pigmentosa. Understanding the function of the Bateman domain in E. coli will shed light on IMPDH’s extracatalytic functions. I constructed E. coli strains that can endogenously express Strep-II tagged IMPDH and IMPDHΔCBS and used affinity purification to test for the presence of binding partners. E. coli cells were harvested during exponential growth and incubated with formaldehyde to chemically cross-link protein- protein and protein-nucleotide interactions prior to lysis. Western blots of IMPDH and IMPDHΔCBS from cross-linked lysates revealed cross-link dependent band shifts that corresponded with the molecular weight of their respective dimer, trimer, and tetramer. There was also a cross-link dependent shift of the wild-type IMPDH monomer that was absent in the IMPDHΔCBS sample, which indicates that the shift was dependent on an interaction with the Bateman domain. Mass spectrometry was inconclusive for determining a protein-binding partner for the Bateman domain, but both the WT tagged and ΔCBS tagged samples contained GMP reductase (GMPR) and adenosine deaminase. Additional research is required to identify the function of these interactions and the molecule(s) that interact with the Bateman domain. iv Table of Contents Acknowledgments………………………………………………………………………………..iii Abstract…………………………………………………………………………………………...iv List of tables……………………………………………………………………………………....vi List of figures………………………………………………………………………………….....vii Introduction………………………………………………………………………………………..1 Materials and methods…………………………………………………………………………….7 Results…………………………………………………………………………………………....18 Discussion………………………………………………………………………………………..31 Appendix I: Common methods…………………………………………………………………..34 Appendix II: Mass spectrometry data….………………………………………………………...40 Appendix III: Materials…………………………………………………………………………..53 References………………………………………………………………………………………..55 v List of Tables 1. Strains……………………………………………………………………………………..7 2. Primers…………………………………………………………………………………….8 3. Plasmids…………………………………………………………………………………...9 4. Concentrations of media additives………………………………………………………...9 5. Mass spectrometry top hits………………………………………………………………27 6. PCR components…………………………………………………………………………35 7. Thermocycler settings for PCR………………………………………………………......35 8. SDS-PAGE recipe………………………………………………………………………..36 9. Complete mass spectrometry data…………………………………………………….....40 vi List of Figures 1. E. coli De novo and salvage pathways of purine biosynthesis……………………………2 2. Bacterial IMPDH homotetramer…………………………………………………………..3 3. Predicted structure of E. coli IMPDH monomer……………………………………….....4 4. λ Red Recombineering System for creating chromosomal mutations…………………...11 5. Tag extension to create ΔCBS tagged strain……………………………………………..12 6. Purification on Strep-Tactin column……………………………………………………..15 7. Western blots confirming the expression of tagged and untagged IMPDH and IMPDHΔCBS………………………………………………………………………………19 8. Growth curves comparing mutant strains to E. coli parent strain………………………..20 9. Luciferase ATP assay…………………………………………………………………....21 10. Western blot from Strep-II tagged IMPDH purification reveals no interaction with RNA polymerase β or Ribosome………………………………………………………………22 11. Western blot from formaldehyde cross-linking of WT tagged lysate...………………….24 12. Western blot from formaldehyde cross-linking of ΔCBS tagged lysate…………………25 13. Western blot with formaldehyde cross-linked lysates…………………………………...26 14. Coomassie-stained SDS-PAGE gel containing elution samples from parent, WT tagged, and ΔCBS tagged………………………………………………………………………...30 vii Introduction Inosine 5’-monophosphate dehydrogenase (IMPDH) converts inosine 5’-monophosphate (IMP) to xanthosine 5’-monophosphate (XMP) as a part of the de novo purine biosynthesis pathway. IMP is a precursor to both adenine and guanine nucleotides and its conversion to XMP by IMPDH is the first committed and rate-limiting step in the biosynthesis of guanosine 5’- monophosphate (GMP). XMP is converted to GMP by GMP synthetase (GMPS). Inhibition of IMPDH results in a depletion of guanine nucleotide pools. The de novo guanine nucleotide synthesis pathway containing IMPDH and GMPS is found in nearly all organisms with the exception of certain protozoan parasites including Giardia lamblia and Trichomonas vaginalis (1,2) and some bacteria. Guanine nucleotides can be produced by salvage pathways in many organisms using phosphoribosyltransferases, nucleoside kinases, or a combination of the two as shown in Figure 1 (3). The rate of nucleotide production by the de novo pathway compared to that of the salvage pathways determines whether or not a given organism or tissue will be susceptible to IMPDH inhibitors. For example, the salvage pathways of E. coli are unable to sustain growth in minimal media when the gene encoding IMPDH, guaB, is deleted from the chromosome. In order to grow in minimal media, the ΔguaB strain must be supplemented with GMP precursors such as xanthosine or guanosine. Guanine nucleotides are vital for many cellular functions including DNA replication, transcription, translation, and as a cofactor for G-proteins. IMPDH is overexpressed in tissues such as tumors because of the high demand for guanine nucleotides in rapidly proliferating cells (4). As a result, inhibitors of IMPDH have been used as anticancer, antiviral, and immunosuppression treatments (5). IMPDH is also required for many microbial pathogens to cause an infection, which suggests that IMPDH inhibitors could be used as antimicrobials (6). 1 NH2 N N N N O HO O NH OH HO O OH OH O O O OH N P P P O O O O Pyrimidines Adenosine 5'-triphosphate HO HO OH NH2 prs uridine N N N N O O O HO O P HO HO O HN N deoD HN N N N HO OH OH H2N N H2N N O O OH O H P P HO O HO guanine O O O OH OH HO P Adenosine 5'-diphosphate O O HO OH P guanosine O OH gpt NH2 PRPP gsk N N N N O O HO O OH N P HN HO OH O N H2N N Adenosine 5'-monophosphate O HO OH O OH P HO purB O guanosine 5'-monophosphate OH GMPR guaC O HO NH OH OH O N N guaA N N N N GMPS purA IMPDH N N N N N HO N O guaB O O HO apt HO HO OH O O O OH O OH P P P HO HO HO HO OH O OH O adenylosuccinate inosine 5'-monophosphate xanthosine 5'-monophosphate gpt gsk O H NH2 OH hpt N NH2 HN H N N N N N N O N N H deoD N N N N N N add Xanthine O O adenine HO HO OH OH HO HO OH Adenosine Inosine deoD deoD N N OH H HO N N N N O N HO N OH Hypoxanthine HO Xanthosine Figure 1: E. coli De novo and salvage
Details
-
File Typepdf
-
Upload Time-
-
Content LanguagesEnglish
-
Upload UserAnonymous/Not logged-in
-
File Pages64 Page
-
File Size-