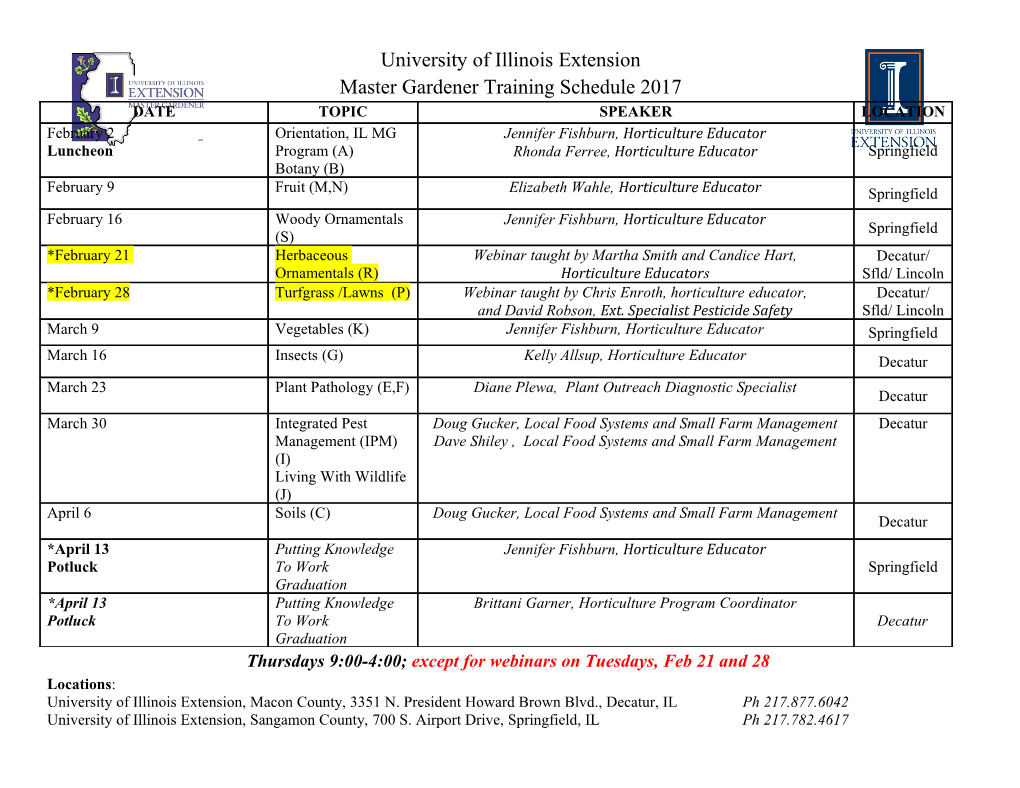
Development of Microstrip-Coupled Lumped-Element Kinetic Inductance Detectors for Astronomy A thesis submitted in partial fulfilment of the requirements for the degree of Doctor of Philosophy by Amber Loren Hornsby November 2020 Abstract Lumped-element Kinetic Inductance Detectors (LEKIDs) are superconducting Inductor- Capacitor (LC) resonators that have been utilised in large-format arrays of detectors for millimetre-wave astronomy. LEKIDs are highly multiplex-able by design and have been demonstrated to reach the photon-noise limit required for astronomy. However, coupling such structures directly to low loss superconducting transmis- sion lines and filtering structures has proved challenging. The research presented in this thesis is aimed at developing techniques for coupling a LEKID to an an- tenna structure and microwave transmission line, which would be compatible with existing device architecture used in existing Cosmic Microwave Background (CMB) experiments. Several important developments towards realising large arrays of multi-chroic, polarisation-sensitive pixels suitable for future Cosmic Microwave Background ex- periments are presented in this thesis. These include coupling a Kinetic Inductance Detector (KID) to an antenna and microstrip, which is made possible by the sepa- rated inductor and capacitor architecture of a LEKID. The results presented in this thesis demonstrate that a dielectric can be deposited over the inductor only, to form a coupling microstrip structure, while leaving the LEKID relatively immune to the noise created by the typically noisy dielectrics. The section of microstrip between the antenna and KID can then be utilised to introduce on-chip filtering structures which limit the band of frequencies reaching the detector. Initial measurements of single-colour prototype devices suggest the current devices are susceptible to stray light, an issue that needs to be considered in future design iterations. However, the overall results presented in this thesis still represent an important step towards real- ising large arrays of multi-chroic, polarisation-sensitive detectors required for future studies of the CMB. i Contents Abstracti List of Figuresv List of Tables viii List of Acronyms ix List of Variables xi Acknowledgements xx 1 Introduction1 1.1 Cosmic Microwave Background ..................... 4 1.1.1 B-modes - Hidden in the Anisotropies.............. 6 1.1.2 Focal-plane Requirements .................... 7 1.2 Kinetic Inductance Detectors ...................... 10 1.3 Summary ................................. 13 1.4 Thesis outline............................... 14 1.5 Literature Contributions......................... 15 2 Physics of Kinetic Inductance Detectors 17 2.1 Normal State Conductivity........................ 18 2.2 London Model............................... 19 2.3 Two-Fluid Model............................. 20 2.4 Mattis-Bardeen Superconductivity.................... 23 2.5 Internal Inductance of a Superconductor ................ 27 2.6 Surface impedance of a Superconducting Film............. 29 2.7 Quasi-particle Dynamics......................... 32 ii Contents 2.7.1 Thermal Excitations ....................... 33 2.7.2 Photon Absorption........................ 34 2.8 Geometry of a KID............................ 35 2.9 Quality Factors.............................. 36 2.10 Impedance of the Resonator....................... 37 2.11 Transmission of Resonator........................ 38 2.12 Summary ................................. 39 3 Response of a LEKID 41 3.1 Modelling a KID ............................. 41 3.1.1 Temperature Response...................... 45 3.1.2 Optical Load ........................... 48 3.2 Noise of a KID .............................. 51 3.2.1 Detector Bandwidth ....................... 51 3.2.2 Generation-Recombination Noise ................ 52 3.2.3 Amplifier Noise.......................... 53 3.2.4 Two-Level Systems........................ 53 3.3 Summary ................................. 54 4 Overview of Detector Arrays 55 4.1 Experimental Techniques......................... 56 4.2 The Dielectric Detectors......................... 58 4.2.1 Device 1.............................. 59 4.3 The Antenna-Coupled Detectors..................... 63 4.3.1 Device 2.............................. 64 4.4 Fabrication ................................ 68 4.5 Summary ................................. 71 5 The Dielectric Requirement 73 5.1 Resonator response............................ 73 5.2 Device 1.................................. 75 5.2.1 Experimental set-up ....................... 76 5.2.2 Dark Response .......................... 77 5.2.3 Dark Detector Noise ....................... 80 iii Contents 5.2.4 Dark Noise Equivalent Power .................. 82 5.3 Summary ................................. 85 6 On-chip filtering structures 87 6.1 Quarter-wave stub filter ......................... 88 6.1.1 ABCD Matrices.......................... 90 6.1.2 Designing a quarter-wave stub.................. 91 6.1.3 Comparison of simulations.................... 95 6.2 Designing multi-pole band-pass filters.................. 96 6.2.1 Broad-band filter......................... 96 6.2.2 Narrow-band filter ........................ 97 6.3 Spectral response............................. 99 6.3.1 No filter..............................100 6.3.2 Broad-band filter.........................101 6.3.3 Narrow-band filter ........................103 6.4 Comparing the results with simulations . 103 6.5 Summary .................................106 7 Optical Results 107 7.1 Device 2..................................107 7.1.1 Experimental set up .......................108 7.1.2 Scaling detector response.....................109 7.1.3 Dark response...........................111 7.1.4 Optical response .........................111 7.2 Aperture testing of Device 2.......................114 7.2.1 Single aperture ..........................117 7.2.2 Multiple apertures ........................118 7.2.3 Impact of detector location....................120 7.3 Simulations ................................123 7.4 Summary .................................125 8 Future Work and Conclusion 127 iv List of Figures 1.1 An ideal multi-chroic, polarisation-sensitive pixel design........ 3 1.2 Planck’s view of the Universe....................... 5 1.3 The history of the Universe........................ 6 1.4 Brightness temperature rms versus f and astrophysical component... 8 1.5 A history of millimetre/sub-millimetre experiments........... 9 1.6 An example of a detector responding to photon absorption. 11 1.7 The circuit diagram for multiplexed, capacitively coupled LEKIDs. 12 2.1 Ratio of population densities of ns and nqp to n............. 21 2.2 A circuit diagram describing the two fluid model. ........... 21 2.3 ∆ as a function T. ............................ 24 2.4 Full Mattis Bardeen calculation of σ1................... 25 2.5 Full Mattis Bardeen calculation of σ2................... 25 2.6 Full and approximation of σ1. ...................... 26 2.7 Full and approximation of σ2. ...................... 26 2.8 Current density of a thick and thin film. ................ 28 2.9 Lk versus T and film thickness. ..................... 30 2.10 Lk and Lm versus T and film thickness.................. 30 2.11 Rs versus T/Tc as a function of f..................... 31 2.12 Xs versus T/Tc as a function of f..................... 31 2.13 Effective T of superconducting volume.................. 33 2.14 σ1/σn versus f for Al and Nb. ...................... 35 2.15 Circuit diagram for a capacitively-coupled LEKID. .......... 36 2.16 Definition of scattering parameters.................... 39 2.17 The S21 transmission of a LEKID..................... 40 3.1 The device layer of a Sonnet simulation of example detector...... 42 v List of Figures 3.2 The groundplane layer of a Sonnet simulation of an example detector. 42 3.3 Calculating Lg from two Sonnet simulations............... 44 3.4 S21, as a function of T for example detector............... 47 3.5 Fractional frequency shift versus T of example resonator. 47 3.6 Nqp versus temperature. ......................... 48 3.7 S21, as a function of T, with optical load................. 50 3.8 Fractional frequency shift due to optical load. ............. 50 4.1 The electronics readout chain for a homodyne readout. 56 4.2 Schematic describing different dielectric scenarios............ 58 4.3 Overall detector layout of the dielectric chip............... 59 4.4 Device layers for device 1......................... 60 4.5 S21 of device 1 from Sonnet simulations and VNA measurements. 61 4.6 Images of the (a) device and (b) lenslet array. ............. 63 4.7 Detector layout of optical chip. ..................... 64 4.8 Device layers for device 2......................... 65 4.9 S21 of device 2 from Sonnet simulations and VNA measurements. 67 4.10 Fabricating a mc-LEKID. ........................ 68 5.1 f versus T for an example resonator.................... 74 5.2 Diagram defining the fill factor...................... 76 5.3 Examples of bifurcation in a KID..................... 77 5.4 Fractional frequency shift of covered resonators............. 78 5.5 Fractional frequency shift of partially-covered resonators. 78 5.6 Fractional frequency shift of bare resonators............... 79 5.7 Fδ0 for each resonator .......................... 79 5.8 Measured noise power spectrum of resonators.............. 81 5.9 Fractional frequency noise at a modulation frequency of 50 Hz. 81 5.10 Estimating the frequency responsivity of the detectors. 83 5.11
Details
-
File Typepdf
-
Upload Time-
-
Content LanguagesEnglish
-
Upload UserAnonymous/Not logged-in
-
File Pages167 Page
-
File Size-