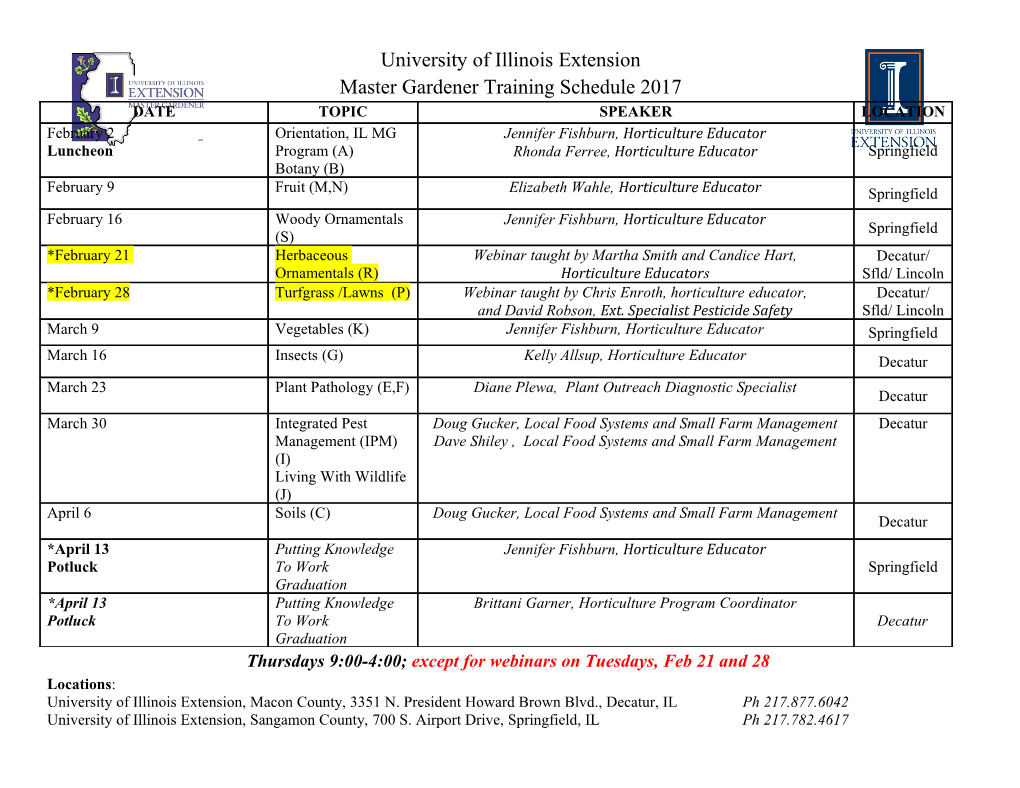
Integrated Violin Pickup, Effects, and Amplifier By Thanh N. Nguyen, Peter Sudermann, and Chetan Sharma 6.101, Spring 2017 Table of Contents Abstract Introduction Project Overview Block Diagram Magnetic Pickup - Thanh Nguyen Objectives High Level Implementation Low Level Implementation Results Preamplification and Tone Control - Thanh Nguyen Objectives Preamplification Implementation Tone Control Implementation Result Effects Stage - Peter Sudermann Objectives High Level Implementation Low Level Implementation Results Amplification Stage - Chetan Sharma Objectives High Level Implementation Low Level Implementation Physical Implementation Results Conclusion Final Project Results Final Thoughts and Insights Citations Abstract This project aimed to create an integrated violin pickup, effects stage, and amplifier. The violin pickup was implemented as a laser-cut humbucker magnetic pickup with a non-inverting preamplifier and a Baxandall tone control network. The effects stage included a tube compressor designed to introduce soft clipping and a spring reverb tank. The final output amplifier tool the form of a PCB mounted Class D amplifier. The resulting system worked as expected with minimal distortion and decent reliability. Modular design and testing, as we learned, were invaluable elements of the design process. Introduction Most musical instruments can have their notes converted into electrical signals; the violin is no exception. Since the 1920s, violins with electric pickups have been used by performers worldwide. Their popularity could be attributed to their versatility; an electric audio signal can be transformed and amplified in fashions that permit unique and novel sounds to be created. This gives the performer a greater variety of tools to create music and perform it in a large concert stage for thousands of people. The goal of this project is to create a complete system capable of picking up notes from a standard violin, applying effects to them, and amplifying them to a level suitable for performance. Notes will be captured using a magnetic pickup. Piezoelectric pickups are traditionally used for violins, but a magnetic pickup has the advantage of depending less on the particular acoustics of the instrument. A preamplifier immediately boosts this incoming signal to operational levels. The captured signal will then be sent to the sound effects stage. The sound effects stage serves to add complexity to the relatively dry, acoustically uninteresting signal of the pickup. This stage makes use of antique analog audio equipment by using a tube compressor and hardware spring reverb. The wet signal will then be sent to the output stage. The output stage of this project takes the form of a Class D amplifier. The Class D architecture allows one to amplify sound accurately without compromising efficiency; a well-made Class D amplifier can achieve efficiencies above 95%. Project Overview Block Diagram Figure 0.1: Block Diagram Magnetic Pickup - Thanh Nguyen Objectives The first block of our project is the magnetic pickup. It creates a signal that copies the sound of the violin. The captured signal is output as a voltage. Additionally, the pickup should only capture the sound from the violin and not any other EM waves from any other sources. The functional requirement of this block is to create at least a 50 mV peak-to-peak signal with noise at least 1/10 the strength of the signal. The challenges of this block are ensuring that the captured signal is strong enough, the common mode noise from stray EM waves is small, and the sound from each string is equally captured. High Level Implementation The magnetic pickup is composed of a magnet and a coil. The pickup is placed underneath the strings of a violin. It’s important to make sure that the strings are magnetically permeable i.e. steel and not gut core. As the string is vibrated to make sound, a small current is induced in the string due to it moving in the magnetic field of the magnetic. The small current induced in the string generates its own magnetic field. Since the string is constantly vibrating to make sound, the generated magnetic field from the moving string is also constantly changing. Since a change in a magnetic field within a coil generate a voltage, the coil produces a voltage that represents the constantly changing magnetic field and thus the sound of the moving steel string (Seering). Figure 1.1: Schematic of a magnetic pickup Photo Credit: audio-pro-central.com Low Level Implementation In the design of the magnetic pickup for the violin, there are three major issues. The first issue is that the magnetic pickup will capture any stray electromagnetic wave especially the 60 Hz AC hum from any outlet. This will induce a constant hum in the signal. To cancel out this common mode noise, we employed a humbucker configuration of coil. Essentially, instead of one big coil capturing the signal, we used two small coils -- one wounded clockwise and one wounded counterclockwise. The common mode noise appears over both coils and is attenuated. However, each individual string produces a local change in magnetic field and is not equally picked up by both coils. Our implementation of the humbucker configuration is shown below in figure 3.2. The violin has four strings. Under string G and D there is one coil wound clockwise. For string A and E, there is another coil underneath wound counterclockwise. Figure 1.2: Top view of the magnetic pickup. The next issue is more of a mechanical issue but is worth noting due to its importance to maintaining sound balance. For the sound to be captured equally on each of the violin string, we need to ensure the magnet can place an equal magnetic field at each of the violin string. However, on a violin, each string has an unequal height to the body of the violin, so a simple bar magnet placed underneath the strings will not work. Figure 1.3: Note the distance of each string to the body of the violin To solve this issue, four threaded standoffs is placed on top of a bar magnet. Each standoff is spaced out similarly to how the strings are spaced out. A bolt is inserted into each of the standoff. It’s important that the standoffs and the bolts are magnetically permeable. The inserted bolt can adjust its own height by screwing down and up the standoff. With this setup, we can transmit the magnetic field from the bar magnet up through the standoffs and bolt. Since the bolt is height adjustable, we can ensure that an equal magnetic field is produced at each string. Figure 1.4: Cross section view of the magnetic pickup The last issue in building the magnetic pickup is self-resonance. The pickup can be modeled as an LRC circuit. The coil is a loop of wires, so it will have inductance. The long length of wire needed to create the coil has resistance. Lastly, the close packing of the coil creates a capacitance. If the resonance is within the hearing frequency (20 - 20kHz), the voltage out would not be balanced across the full range of the violin. There would be frequencies where the sound would be louder and other softer. This brings forth a design constraint with the pickup. The pickup cannot just have a lot of windings to have a big output, but it also has to have a small enough amount of windings to create a self resonance outside the hearing frequency. In order to arrive at the correct amount of windings, an iterative process must be taken, but due to a time constraint, the amount of winding is decided by mimicking the design of another magnetic pickup (Jenn). The final design uses a N52 grade neodymium bar magnet and two coils of 4000 turns. Figure 1.5: Circuit model of the magnetic pickup Figure 1.6: Top view of the magnetic pickup. Figure 1.7: Side view of the magnetic pickup. Results From a rough hearing test, the higher frequencies, playing on the E string of the violin, have a slight roll off in volume. It’s noticeable softer than sounds played on the G, D, and A strings (lower frequency). The output signal at a medium playing level is 200 mV peak-to-peak. Although untuned correctly, the next stage, the preamplifier, will help with equalizing the signal. During the winding process, we also accidentally missed counted, making one coil about 500 turns higher than the other one. Thus, we did not perfectly attenuated the 60 Hz AC hum. The noise signal is measured at 7 mV peak-to-peak in a normal room environment. However, the noise signal can get as high as 50 mV peak-to-peak when the magnetic pickup is near a transformer. Preamplification and Tone Control - Thanh Nguyen Objectives The next step in our project is to amplify and filter the signal produced by the magnetic pickup. The output of the magnetic pickup is roughly 200 mVpp. We want to boost this signal up to line level which is 1 Vrms as the first functional requirement for this block. We want to filter the signal to only capture the signal from the hearing frequencies. Lastly, we want to give the performer more control over their instrument, so we will also have a tone control as the second functional requirement. It will be used to attenuate or boost the signal of the bass frequencies (10 Hz - 1 kHz) and the treble frequencies (1 kHz - 20 kHz). Figure 2.1: Overall schematic of this block Preamplification Implementation Figure 2.2: First Preamplifier The preamplification circuit is very simple. It’s just an op-amp in a non-inverting amplifier configuration with some capacitors to act as filters.
Details
-
File Typepdf
-
Upload Time-
-
Content LanguagesEnglish
-
Upload UserAnonymous/Not logged-in
-
File Pages31 Page
-
File Size-