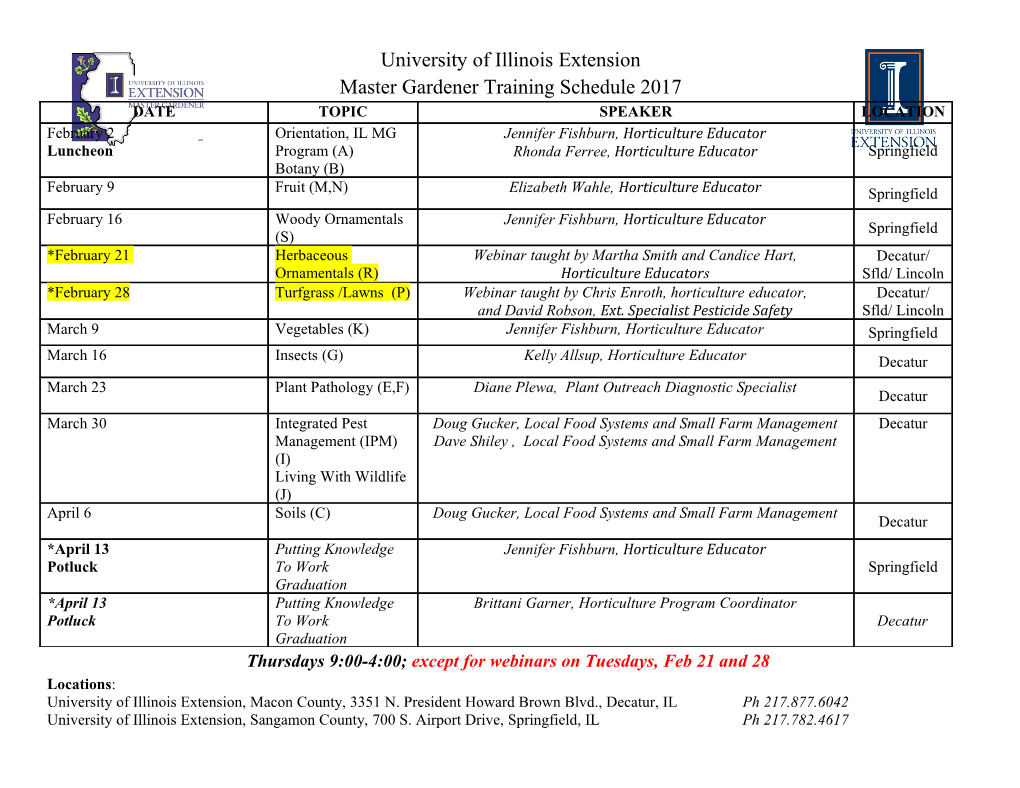
bioRxiv preprint doi: https://doi.org/10.1101/2021.02.24.432815; this version posted February 25, 2021. The copyright holder for this preprint (which was not certified by peer review) is the author/funder, who has granted bioRxiv a license to display the preprint in perpetuity. It is made available under aCC-BY 4.0 International license. 1 SIRT1 regulates sphingolipid metabolism and neural 2 differentiation of mouse embryonic stem cells through c-Myc- 3 SMPDL3B 4 5 Wei Fan,1 Shuang Tang, 1, 6 Xiaojuan Fan, 2 Yi Fang,1 Xiaojiang Xu, 3 Leping Li, 4 Jian Xu, 6 5 Jian-Liang Li, 3 Zefeng Wang, 2, * and Xiaoling Li 1, * 7 8 1Signal Transduction Laboratory, National Institute of Environmental Health Sciences, 9 Research Triangle Park, NC 27709, USA 10 2CAS Key Laboratory of Computational Biology, CAS-MPG Partner Institute for 11 Computational Biology, Shanghai Institute of Nutrition and Health, Shanghai Institutes for 12 Biological Sciences, University of Chinese Academy of Sciences, CAS Center for 13 Excellence in Molecular Cell Science, Chinese Academy of Sciences, Shanghai 200031, 14 China 15 3Integrative Bioinformatics Support Group, National Institute of Environmental Health 16 Sciences, Research Triangle Park, NC 27709, USA 17 4Biostatistics & Computational Biology Branch, National Institute of Environmental Health 18 Sciences, Research Triangle Park, NC 27709, USA 19 5Children's Medical Center Research Institute, Department of Pediatrics, Harold C. 20 Simmons Comprehensive Cancer Center, and Hamon Center for Regenerative Science 21 and Medicine, University of Texas Southwestern Medical Center, Dallas, TX 75390, USA. 22 6Current address: Cancer Institute and Department of Nuclear Medicine, Fudan University 23 Shanghai Cancer Center, Shanghai 200032, China 24 25 1 bioRxiv preprint doi: https://doi.org/10.1101/2021.02.24.432815; this version posted February 25, 2021. The copyright holder for this preprint (which was not certified by peer review) is the author/funder, who has granted bioRxiv a license to display the preprint in perpetuity. It is made available under aCC-BY 4.0 International license. 26 * Correspondence: [email protected] (X. L.), [email protected] (Z. W.) 27 Short title: SIRT1 regulates sphingolipid metabolism 28 Key words: SIRT1; sphingomyelin degradation; embryonic stem cells; neural development; 29 embryogenesis; c-Myc 30 2 bioRxiv preprint doi: https://doi.org/10.1101/2021.02.24.432815; this version posted February 25, 2021. The copyright holder for this preprint (which was not certified by peer review) is the author/funder, who has granted bioRxiv a license to display the preprint in perpetuity. It is made available under aCC-BY 4.0 International license. 31 Abstract 32 Sphingolipids are important structural components of cell membranes and 33 prominent signaling molecules controlling cell growth, differentiation, and apoptosis. 34 Sphingolipids are particularly abundant in the brain, and defects in sphingolipid 35 degradation are associated with several human neurodegenerative diseases. However, 36 molecular mechanisms governing sphingolipid metabolism remain unclear. Here we 37 report that sphingolipid degradation is under transcriptional control of SIRT1, a highly 38 conserved mammalian NAD+-dependent protein deacetylase, in mouse embryonic stem 39 cells (mESCs). Deletion of SIRT1 results in accumulation of sphingomyelin in mESCs, 40 primarily due to reduction of SMPDL3B, a GPI-anchored plasma membrane bound 41 sphingomyelin phosphodiesterase. Mechanistically, SIRT1 regulates transcription of 42 Smpdl3b through c-Myc. Functionally, SIRT1 deficiency-induced accumulation of 43 sphingomyelin increases membrane fluidity and impairs neural differentiation in vitro and 44 in vivo. Our findings discover a key regulatory mechanism for sphingolipid homeostasis 45 and neural differentiation, further imply that pharmacological manipulation of SIRT1- 46 mediated sphingomyelin degradation might be beneficial for treatment of human 47 neurological diseases. 48 49 50 51 3 bioRxiv preprint doi: https://doi.org/10.1101/2021.02.24.432815; this version posted February 25, 2021. The copyright holder for this preprint (which was not certified by peer review) is the author/funder, who has granted bioRxiv a license to display the preprint in perpetuity. It is made available under aCC-BY 4.0 International license. 52 Introduction 53 First isolated from brain extract in the 1880s, sphingolipid is a class of natural lipids 54 containing a backbone of sphingoid base sphingosine (1-3). Sphingolipids not only are the 55 structural components of cell membranes, but also act as signaling molecules to control 56 various cellular events, including signal transduction, cell growth, differentiation, and 57 apoptosis (4, 5). Particularly, sphingolipids are enriched in microdomains/lipid rafts, a 58 liquid-ordered phase in plasma membrane, where sphingomyelin, glycosphingolipids and 59 cholesterol form unique platforms for many different proteins that are important for nutrient 60 transport, organelle contact, membrane trafficking, and homotypic fusion (6). 61 The homeostasis of sphingolipids is maintained by a highly coordinated metabolic 62 network that links together various pathways with ceramide as a central node (Figure S1A). 63 Firstly, ceramide can be de novo synthesized in endoplasmic reticulum (ER), starting from 64 the condensation of serine and fatty acyl-CoA by serine palmitoyltransferase (SPT). 65 Ceramide is then transported to the Golgi complex and further converted into more 66 complex forms of sphingolipids, such as glycosphingolipids or sphingomyelin. Secondly, 67 ceramide can be regenerated by hydrolysis of complex sphingolipids in the Golgi complex 68 and plasma membrane, which is catalyzed by a class of specific hydrolases and 69 phosphodiesterases. For example, regeneration of ceramide from sphingomyelin can be 70 mediated by plasma membrane bound sphingomyelin phosphodiesterases (SMPDs), 71 including SMPDL3B, a GPI-anchored lipid raft SMPD (7). By degrading sphingomyelin, 72 SMPDL3B regulates plasma membrane fluidity, which in turn impacts TLR-mediated 73 innate immunity in macrophages (7) and modulates insulin receptor signaling in podocytes 74 (8). Sphingolipid metabolism is highly sensitive to environmental/nutritional perturbations. 75 Sphingolipid biosynthesis and accumulation can be induced by high-fat diet (HFD) feeding 76 in multiple tissues in mice (9). Sphingolipids are also accumulated during aging (10, 11), 77 and caloric restriction, a dietary regimen known to extend life span and delay a number of 4 bioRxiv preprint doi: https://doi.org/10.1101/2021.02.24.432815; this version posted February 25, 2021. The copyright holder for this preprint (which was not certified by peer review) is the author/funder, who has granted bioRxiv a license to display the preprint in perpetuity. It is made available under aCC-BY 4.0 International license. 78 age-associated diseases, decreases sphingolipid accumulation by reducing the activity of 79 SPT (12). Dietary serine restriction also alters sphingolipid diversity and synthesis to 80 constrain tumor growth (13). 81 Disruption of sphingolipid homeostasis has been involved in the pathogenesis of 82 a number of human diseases, such as Niemann-Pick disease and neurodegenerative 83 Alzheimer’s, Parkinson’s, and Huntington’s diseases. These human diseases are 84 generally the outcome of defect in enzymes that degrade the sphingolipids (14, 15). Such 85 degradation defect leads to accumulation of sphingolipids, which in turn dramatically alters 86 cellular membrane structure and signaling, thereby triggering various diseases. 87 Consequently, manipulation of one enzyme or metabolite in sphingolipid degradation 88 pathways may result in unexpected changes in cellular metabolic programs and related 89 cellular functions (14, 15). However, molecular mechanisms that regulate the expression 90 of these sphingolipid degrading enzymes remain unclear. 91 In the present study, we investigated the role of SIRT1 in regulation of sphingolipid 92 degradation in mouse embryonic stem cells (mESCs) and mouse embryos. SIRT1 is a 93 NAD+-dependent protein deacetylase critical for multiple cellular processes, including 94 metabolism, inflammation, stress response, and stem cell functions (16-18). SIRT1 is also 95 a key regulator of animal development (19-21), and is particularly important in the central 96 nervous system. For instance, SIRT1 has a major influence on hypothalamic function, and 97 cell-type specific SIRT1 mutations result in defects in systemic energy metabolism, 98 circadian rhythm, and the lifespan of the animal (22-25). SIRT1 also modulates dendritic 99 and axonal growth (26, 27), and regulates synaptic plasticity and memory formation in 100 adult brain (28). Moreover, SIRT1 has been shown to ameliorate neurodegenerative 101 phenotypes in animal models of Alzheimer’s, Parkinson’s, and Huntington’s disease 102 (reviewed in (29)). However, despite multiple mechanisms proposed for these critical roles 103 of SIRT1 in the brain, how SIRT1 regulates neural development and function remain 5 bioRxiv preprint doi: https://doi.org/10.1101/2021.02.24.432815; this version posted February 25, 2021. The copyright holder for this preprint (which was not certified by peer review) is the author/funder, who has granted bioRxiv a license to display the preprint in perpetuity. It is made available under aCC-BY 4.0 International license. 104 unclear. Through global metabolomics and cellular
Details
-
File Typepdf
-
Upload Time-
-
Content LanguagesEnglish
-
Upload UserAnonymous/Not logged-in
-
File Pages49 Page
-
File Size-