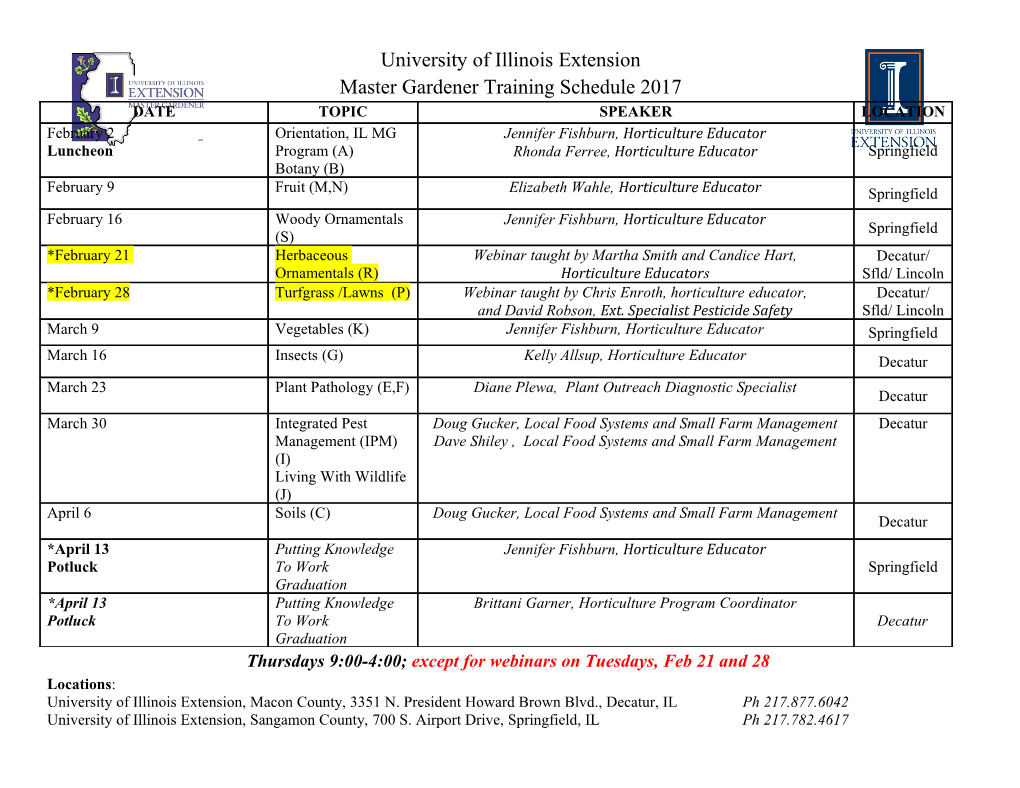
PDF hosted at the Radboud Repository of the Radboud University Nijmegen The following full text is a publisher's version. For additional information about this publication click this link. http://hdl.handle.net/2066/65589 Please be advised that this information was generated on 2021-09-24 and may be subject to change. Targeting the dosage compensation complex to the male X chromosome of Drosophila melanogaster Targeting the dosage compensation complex to the male X chromosome of Drosophila melanogaster Gene Expression programme European Molecular Biology Laboratory (EMBL) Heidelberg, Germany And Department of Molecular Biology Nijmegen Centre for Molecular Life Sciences (NCMLS) Radboud University Nijmegen, The Netherlands Published by the Radboud University Nijmegen Printed by Ponsen & Looijen bv, Wageningen ISBN/EAN: 978-90-9023194-5 Cover: Artistic interpretation of a Drosophila polytene chromosome squash Targeting the dosage compensation complex to the male X chromosome in Drosophila melanogaster Een wetenschappelijke proeve op het gebied van de Natuurwetenschappen, Wiskunde en Informatica Proefschrift ter verkrijging van de graad van doctor aan de Radboud Universiteit Nijmegen op gezag van de rector magnificus prof. mr. S.CJ.J. Kortmann volgens besluit van het College van Decanen in het openbaar te verdedigen op vrijdag 20 juni 2008 om 10.30 uur precies door Jop Haico Kind geboren op 28 december 1978 te Lelystad Promotor: prof. dr. H.G. Stunnenberg Copromotor: dr. A. Akhtar, European Moleculair Biology Laboratory Heidelberg, Duitsland Leden manuscriptcommissie: prof. dr. G.J.M Martens prof. dr. C.P. Verrijzer, Erasmus Universiteit Rotterdam dr. B. van Steensel, Nederlands Kanker Instituut Amsterdam “Caminantes, no hay caminos, hay que caminar” (Text found at a monastery in Toledo, Spain) Table of contents Chapter 1 General introduction 09 preview 10 chromatin 10 higher order chromatin and structure 11 epigentics 13 remodeling chromatin 14 nucleosome assembly/disassembly 15 atp-dependent chromatin remodeling 16 histone variants 18 post translational modifications of histones 19 histone code 21 ptms and chromatin structure 23 thinking globally: the nuclear architecture 25 gene anchoring to the nuclear periphery 26 targeting genes to the npc by mlp1 and mlp2 27 dosage compensation 27 targeting the dosage compensation complex to the x chromosome 29 aim o f the study 30 Chapter 2 Cotransciptional recruitment of the dosage compensation complex to 41 X-linked target genes Chapter 3 Genome-wide analysis reveals MOF as a key regulator of dosage 69 compensation and gene expression in Drosophila Chapter 4 Nuclear pore components are involved in the transcriptional regulation 123 of dosage compensation in Drosophila Chapter 5 Summary and general discussion 159 Acknowledgements 169 Curriculum Vitae 171 List of publications 173 General Introduction 10 Chapter 1 General Introduction Preview Chromosomes in the nucleus of cells contain all the information a living organism needs to carry out its life processes. Chromosomes are made up of four different nucleotides. These four molecules are comprised of a deoxyribose backbone with one of four (adenine (A), cytosine (C), guanine (G), thymine (T)) nitrogenous bases attached. Different combinations of these four bases make up genes, which encode proteins, the building blocks required to “run” a cell. Each human cell contains about 6 billion base-pairs, which equals about 2 meters in length. This poses two problems: 1) How does two meters of DNA fit in a 5-20 micrometer (a millionth of a meter) large nucleus? 2) If all cells have the same material, how does the one cell becomes muscle, whereas another cell constitutes an eyelash? The answer, which also happened to be the subject of my thesis, is chromatin. Chromatin The fundamental unit of chromatin is the nucleosome. Nucleosomes constitute the basic unit of chromatin. One nucleosome is made up of 8 histone proteins, two copies of each H2A, H2B, H3 and H4, around which are wrapped 147 base pairs of DNA, in 1 3/4 left-handed superhelical turns (Finch et al., 1977). In mild physiological conditions, histone H3 and H4 form a tetramer that lays at the center of the nucleosome with a H2A and H2B dimer at either side (Klug et al., 1980; Arends et al., 1991). The nucleosome plus the fifth histone H1, has been termed the chromatosome (for a review see Kornberg, 1999). Only one copy of histone H1 associates with the nucleosome and its association is not required for its constitution (Kornberg 1974). Binding of H1 to both the major groove of the nucleosome dyad and the minor groove of the linker DNA protects another 20bp of DNA (beyond the core particles 147bp) against micrococcal nuclease digestion (Noll and Kornberg 1977; Simpson 1978). The linker DNA is the “free” DNA of variable length between two nucleosomes (Spadafora et al., 1976). Based on the formation of what has been called “superstructures” upon addition of H1 to the nucleosome, H1 is believed to stabilize General Introduction 11 the nucleosome and to facilitate the formation of higher order chromatin structures (Thoma et al., 1979; Ramakrishnan et al., 1997; Bednar et al., 1998). Higher order chromatin structures In cells chromatin comes in two varieties, euchromatin and heterochromatin. Euchromatin is considered an open chromatin structure, whereas heterochromatin represents a highly condensed inaccessible state. Both states can be defined by the presence of distinct chromatin interacting proteins and posttranslational modifications (PTMs) (for a review see Huisinga et al., 2006). Euchromatin is also regarded as active chromatin with most genes and regulatory regions found in euchromatic regions. Heterochromatin is characteristic of gene-poor regions such as telomeres and centromeres. One can visualize heterochromatin by its distinctive bright staining with interchelating dyes such as DAPI, or by its high electron density with electron microscopy. The structure of heterochromatin is believed to be packaged in a compact regular 30nm fiber (see below) whereas euchromatin has a more discontinues nature as judged by its slower sedimentation speed in sucrose gradients (Gilbert and Allan, 2001). Wrapping DNA in nucleosomes is the first degree of compaction. The second step in chromatin condensation is the less characterized 30nm fiber formation. The 30nm fiber is composed of a tandom array of nucleosomes folded in a condensed higher order structure (Gilbert and Rasahoye, 2005). Formation of the fiber is believed to involve stabilization of the linker histone H1 and the unstructured N- terminal tails that are found to stick out from the nucleosome particle (Luger et al., 1997). An interaction has been observed between the N-terminal tail of histone H4 with the flat face surface of the H2A-H2B histone dimer of an adjacent nucleosome, suggestive of a role of this tail in across nucleosome higher-order chromatin formation (Schwartz et al., 1996; Luger et al.,1997). Interestingly, in line with this observation, fully compacted chromatin fibers can be obtained with any of the histone tails deleted except for the histone H4 N-terminus. More specifically the interaction is mediated by an interaction of the positively charged lysine-rich residues 14-19 with negatively charged acidic residues on the H2A-H2B dimer coined the “acidic patch” (Luger et al., 1997; Suto et al., 2000; Dorigo et al., 2003). The importance of this 12 Chapter 1 interaction was further highlighted by the finding that eukaryotic cells have devised a way to change the nature of the acidic patch, by the incorporation of the histone variants H2A.Z and H2ABbd (Bar body deficient). H2A.Z increases, whereas H2Abbd reduces the acidic character of the acidic patch thereby respectively stabilizing and destabilizing the inter-nucleosome interactions (Clakson et al., 1999; Chatwick and Willard 2001 and discussed below). As the N-terminal histone tails are susceptible to a wide range of covalent PTMs, another way of changing this interaction is by neutralization of the positive charge on the H4 N-tail by acetylation (discussed below). Normally interphase cellular chromatin is found as a 30nm fiber (Langmore and Schutt, 1980), Originally the histone linker H1 was placed on the inside of the 30nm fiber with the linker DNA connecting two consecutive nucleosomes within the same stack. Per turn 6-8 nucleosomes coil around a central cavity constituting the superhelix. The formation of such a superhelix is termed a one-start helix (Widom and Klug, 1985). In contrast, in the two-start model the linker DNA is found on the outside of the superhelix connecting nucleosomes of consecutive stacks (Woodcock et al., 1984). Recently, an elegant study showed that most likely the 30nm fiber forms a two-step helix (Dorigo et al., 2004). The authors use the recently identified interaction between the acidic patch and the histone H4 tail to select a pair of cystein- replacement mutants that would allow stabilization (by di-sulfite bridges) of the 30nm fiber. Such a fiber was prone to digestion by a specific restriction enzyme at a site present in the linker DNA, showing that the linker-DNA is found on the outside of the superhelix. Moreover, restriction of the linker DNA of a 10 or 12 nucleosomal repeats resulted in two products of 5 or 6 nucleosomes respectively, explanatory of a two step model with stacks formed by disulfite cross-links and linker DNA connecting nucleosomes of two adjacent stacks (Dorigo et al., 2004). Although at low-angle-X-ray diffraction most of the chromatin appears as 30nm fibers, larger structures of 60-130nm fibers have also been observed by EM. These structures, (termed “chromonema”) are actual fibers most probably comprised of higher order compaction of 30nm fibers (Belmont and Bruce, 1994). The authors estimate that the formation of these “chromonema” results in a 160-1000 compaction. Maximum compaction the two meters of DNA (in interphase cells) thus will result in 2000^m of “chromonema” to fit in a 2-20^m small nucleus.
Details
-
File Typepdf
-
Upload Time-
-
Content LanguagesEnglish
-
Upload UserAnonymous/Not logged-in
-
File Pages171 Page
-
File Size-