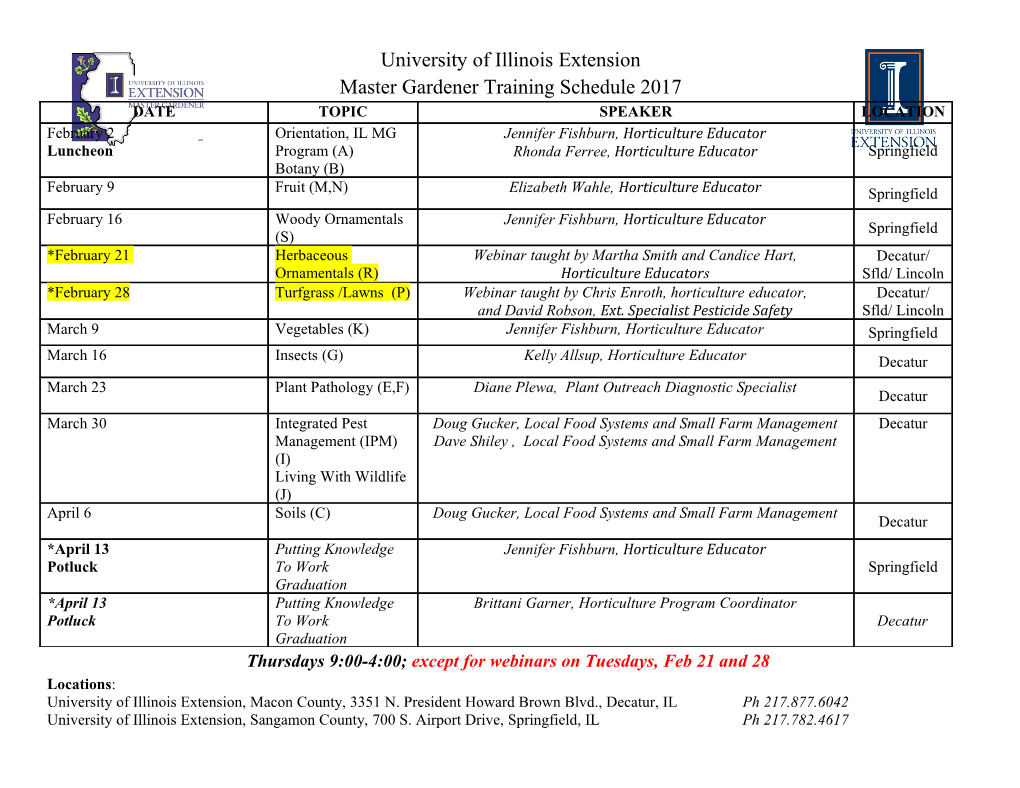
This is the peer-reviewed, final accepted version for American Mineralogist, published by the Mineralogical Society of America. The published version is subject to change. Cite as Authors (Year) Title. American Mineralogist, in press. DOI: https://doi.org/10.2138/am-2021-7415. http://www.minsocam.org/ 1 Revision 1 2 3 Targeting Mixtures of Jarosite and Clay Minerals for Mars 4 Exploration 5 6 Nancy W. Hinman*a, Janice L. Bishopb,c, Virginia C. Gulick b,c, J. Michelle Kotler Dettmanna,1, 7 Paige Morknerd,2, Genesis Berlangac,d,3, Ruth M. Hennebergere,4, Peter Bergquistf, Charles Doc 8 Richardsona,5, Malcolm R. Walterg, Lindsay A. MacKenziea,6, Roberto P. Anitorie,7, Jill R. Scotti 9 10 a Department of Geosciences, 32 Campus Dr., MC 1296, University of Montana, Missoula, MT 11 USA 59812-1296; [email protected] 12 b The SETI Institute, Mountain View, CA USA 94043 13 c NASA Ames Research Center, Moffett Field, CA USA 94035 14 d NASA Internship Program, NASA Ames Research Center, Moffett Field, CA 94035 15 e Department of Chemistry and Biomolecular Science, Macquarie University, North Ryde 2109, 16 NSW, Australia; 17 f Department of Molecular Medicine and Pathology, University of Auckland School of Medicine, 18 Auckland, New Zealand 19 g Australian Centre for Astrobiology, University of New South Wales, Sydney, 2052, Australia. 20 h Department of Biology, University of Portland, OR USA 97203-5798 21 i Chemical Sciences, Idaho National Laboratory, Idaho Falls, ID 83415 22 1 Always consult and cite the final, published document. See http:/www.minsocam.org or GeoscienceWorld This is the peer-reviewed, final accepted version for American Mineralogist, published by the Mineralogical Society of America. The published version is subject to change. Cite as Authors (Year) Title. American Mineralogist, in press. DOI: https://doi.org/10.2138/am-2021-7415. http://www.minsocam.org/ 23 1 Present address: European Space Agency ECSAT, Fermi Avenue, Harwell Campus, Didcot, 24 United Kingdom. 25 2 Present address: ORISE Fellow, National Energy Technology Laboratory, Albany, OR, USA 26 3 Blue Marble Space Institute of Science at NASA Ames 27 4 Present address: Institute of Molecular Health Sciences, Swiss Federal Institute of Technology 28 (ETH), 8093 Zürich, Switzerland 29 5 Present address: NewFields, Helena, MT USA 30 6 Present address: Eastern Washington State University, Cheney, WA USA 31 7 Present address: Biology Department, Clark College, Vancouver, WA USA 32 *Corresponding author 33 Keywords: Mars, surface; Astrobiology; Exobiology; Spectroscopy 34 Running Title: Jarosite Mixtures for Mars Exploration 2 Always consult and cite the final, published document. See http:/www.minsocam.org or GeoscienceWorld This is the peer-reviewed, final accepted version for American Mineralogist, published by the Mineralogical Society of America. The published version is subject to change. Cite as Authors (Year) Title. American Mineralogist, in press. DOI: https://doi.org/10.2138/am-2021-7415. http://www.minsocam.org/ 35 Abstract 36 37 Terrestrial thermal environments can serve as analogs for subsurface environments in the 38 search for life because they regularly host microbial communities, which may leave behind 39 biosignatures. This study focused on an acid-sulfate hydrothermal site as an analog for a 40 potentially habitable environment on Mars. A weathered boulder in the thermal area was 41 dissected, revealing an interior marked with disconnected horizons of differently colored 42 materials, very low pH, and increasing temperature. The mineralogy comprised weathering 43 products from andesite (kaolinite, quartz, clinoptilolite) along with sulfate salts (alunite, jarosite, 44 tschermigite, and copiapite) formed by oxidation of sulfide and ferrous iron. Characterization of 45 organic matter in this boulder and several soil samples yielded interesting but surprising results. 46 Both mass spectrometry and Raman spectroscopy identified organic compounds in portions of 47 the soils and the boulder. Jarosite-associated samples showed more numerous and diverse 48 organic signatures than did Al-bearing silicate samples, despite the lower total organic carbon 49 content of the jarosite-associated soils (0.69 ± 0.07 wt% Corg) compared to the Al-bearing 50 samples (1.28 ± 0.13 wt% Corg). Results from our geochemical, mineralogical, and spectroscopic 51 study of hydrothermal alteration products and salts inform the heterogeneous distribution of 52 inorganic and organic materials that could delineate habitats and demonstrate the limits on 53 organic matter detectability using different analytical techniques. Further, we relate our 54 measurements and results relate directly to current and upcoming martian missions, and we 55 provide recommendations for detection and characterization of minerals and organics as 56 biosignatures on Mars using instruments on future missions. 57 3 Always consult and cite the final, published document. See http:/www.minsocam.org or GeoscienceWorld This is the peer-reviewed, final accepted version for American Mineralogist, published by the Mineralogical Society of America. The published version is subject to change. Cite as Authors (Year) Title. American Mineralogist, in press. DOI: https://doi.org/10.2138/am-2021-7415. http://www.minsocam.org/ 58 1 Introduction 59 60 Multiple geologic environments with evidence of aqueous alteration processes have been 61 identified on Mars (e.g., Bibring et al., 2006; Murchie et al., 2009; Ehlmann and Edwards, 2014). 62 Carter et al. (2013) documented numerous sites on the surface of Mars containing hydrous 63 minerals, including phyllosilicates and sulfate minerals, over the surface of Mars using data from 64 the Observatoire pour la Minéralogie, l'Eau, les Glaces et l'Activité) (OMEGA) and Compact 65 Reconnaissance Imaging Spectrometer for Mars (CRISM) data. Most sites are located in ancient 66 outcrops in Noachian and Hesperian terrains. The recent selection of Jezero crater, which has 67 been interpreted as an episodic paleolake, delta, and/or fluvial plain; Ehlmann et al., 2008; 68 Goudge et al., 2015a,b) as the target for the Mars 2020 landing site emphasizes the relevance of 69 understanding the potential for biosignature preservation in rocks containing carbonates, sulfates, 70 and clay minerasl in the search for evidence of life. Jezero crater contains a volcanic unit dated at 71 ~3.45 Ga that embays the sedimentary fan unit to be investigated by Mars 2020 (Goudge et al., 72 2015a). Volcanic heat in this region likely produced hydrothermal activity, which could have led 73 to the formation of acid-sulfate thermal environments given the acidic nature of volcanic gases. 74 The Mars 2020 rover may be able to identify acid-sulfate minerals, such as jarosite 75 (K(Fe3(SO4)2(OH)6) and other sulfates, that are not observed from orbit. Terrestrial acid-sulfate 76 environments may closely resemble those on early Mars (Nna-Mvondo and Martinez-Frias, 77 2007). Unfortunately, the conditions that could best preserve evidence of ancient life on Mars 78 remain confounding and unclear to the scientific community, and thus additional studies in this 79 area are needed (Walter and Des Marais, 1993, 1999; Farmer and Des Marais, 1999; Des Marais, 80 2001, 2008; Summons et al., 2011). 4 Always consult and cite the final, published document. See http:/www.minsocam.org or GeoscienceWorld This is the peer-reviewed, final accepted version for American Mineralogist, published by the Mineralogical Society of America. The published version is subject to change. Cite as Authors (Year) Title. American Mineralogist, in press. DOI: https://doi.org/10.2138/am-2021-7415. http://www.minsocam.org/ 81 Retention and detection are both important to the prospect of finding organic matter 82 and/or biosignatures during any surface mission. Minerals that retain organic matter or that 83 promote its detection are of value, not only to the identification of organic matter, but to the 84 prospects of finding potential biosignatures in any surface mission. Indeed, acid-sulfate 85 terrestrial hot springs remain high priority analog sites to better interpret the results of future 86 Mars missions (Fairen et al 2010; Martins et al., 2017). 87 On Earth, jarosite forms in sulfate-rich environments where Fe(II) is oxidized. For 88 example, jarosite and related sulfates form in acidic waters associated with sulfide oxidation (e.g., 89 Burns 1987; Alpers et al., 1989; Bigham and Nordstrom, 2000; Jones and Renaut, 2007), in acid 90 sulfate soils near volcanic vents (e.g., Zimbelman et al., 2005; Schiffman et al., 2006; Bishop et al., 91 2007), and where SO2 has oxidized, i.e. at the interface of Fe(II)-bearing rocks and sulfate-rich 92 waters, such as basalt surfaces in contact with oxidizing fluids (e.g., Golden et al., 2005; 93 Chemtob et al., 2010; Schaef et al., 2014). Synthetic jarosite phases are produced in laboratory 94 experiments abiotically at very high temperatures (e.g., Kotler et al. 2008) and biotically at lower 95 temperatures. Khoshkhoo et al. (2015) found that jarosite formed in experiments designed to 96 compare abiotic leaching and bioleaching of copper from sulfide ores. Additionally, jarosite 97 formed in bioleaching experiments in which hyperthermophilic iron oxidizing archaea 98 (Acidianus, Metallosphaera, and Sulfolobus) oxidized Fe(II) in the presence of sulfuric acid at 99 elevated temperature (70°C) (Kaksonen et al. (2016)). We can conclude that biological processes 100 obviate the need for the high temperature
Details
-
File Typepdf
-
Upload Time-
-
Content LanguagesEnglish
-
Upload UserAnonymous/Not logged-in
-
File Pages64 Page
-
File Size-