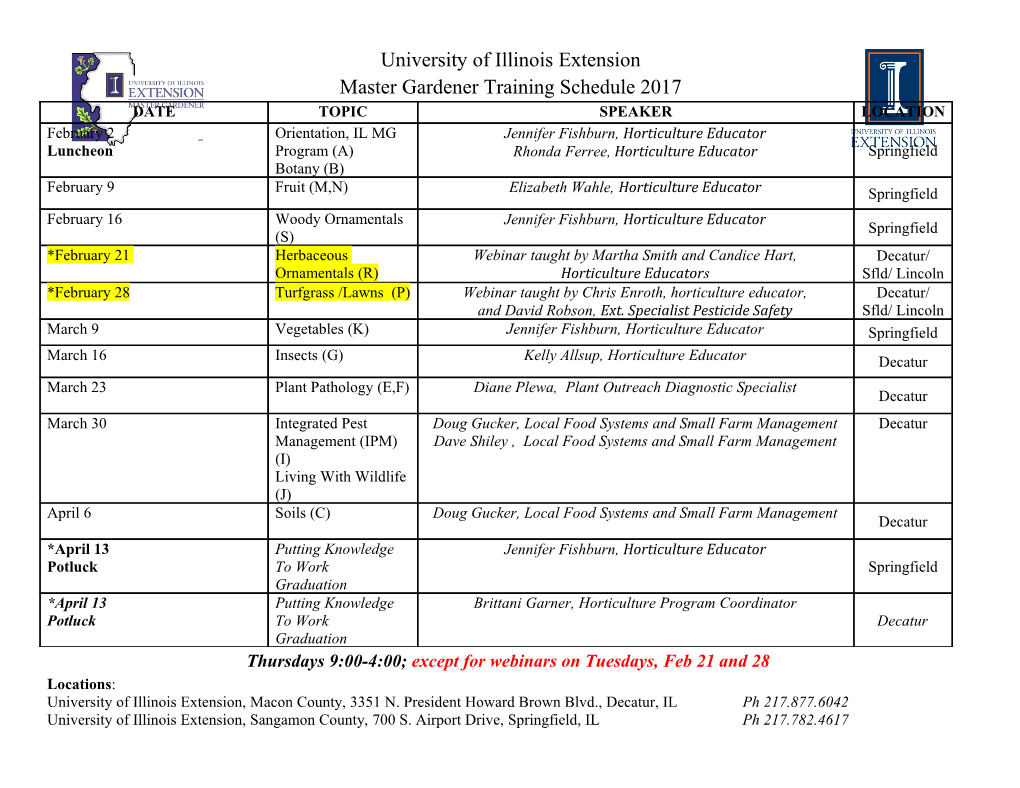
Journal of Nuclear Medicine, published on August 28, 2020 as doi:10.2967/jnumed.120.241984 Quo Vadis Molecular Imaging? Jan Grimm1,2*, Fabian Kiessling3,4*, Bernd J. Pichler5,6* (*authors contributed equally and are listed in alphabetical order) 1Molecular Pharmacology Program & Department of Radiology Memorial Sloan-Kettering Cancer Center, New York, NY, USA 2Pharmacology Program & Department of Radiology, Weil Cornell Medical College, New York, NY, USA 3Institute for Experimental Molecular Imaging, University Hospital Aachen, RWTH Aachen University, Center for Biohybrid Medical Systems (CBMS), Forckenbeckstr. 55, 52074 Aachen, Germany 4Fraunhofer Institute for Digital Medicine, MEVIS, Am Fallturm 1, 28359 Bremen, Germany 5Werner Siemens Imaging Center, Department of Preclinical Imaging and Radiopharmacy, Eberhard Karls University, Tuebingen, Germany 6Cluster of Excellence iFIT (EXC 2180) "Image-Guided and Functionally Instructed Tumor Therapies", University of Tübingen, Germany Corresponding authors: Jan Grimm: Molecular Pharmacology Program, Memorial Sloan Kettering Cancer Center, 1275 York Avenue, New York, NY 10065, USA; phone: +1-646-888-3191; [email protected] Fabian Kiessling: Institute for Experimental Molecular Imaging, RWTH Aachen University, Forckenbeckstrasse 55, D-52074 Aachen; phone: +49-241-8080116; fax: +49-241-803380116; [email protected] Bernd Pichler: Werner Siemens Imaging Center, Department of Preclinical Imaging and Radiopharmacy, Röntgenweg 13, 72076 Tübingen, Germany, phone: +49-7071-29- 83427; [email protected] 1 Abstract Molecular Imaging (MI) yields important insights into relevant biological signatures at an organ-specific and systemic level, which is not achievable with conventional imaging methods and thus provides an essential link between preclinical and clinical research. In this context, new diagnostic probes and imaging methods, revealing comprehensive functional and molecular information, are being provided by MI research, several of which have found their way into clinical application. However, there are also reservations about the impact of MI and its added value over conventional, often less expensive, diagnostic imaging methods. This perspective discusses seminal research directions for the MI field which have the potential to result in added value to the patient. In this context, emphases are set on MI (i) without probes, (ii) based on radiotracers and small molecules, (iii) nano- and microsystems, and (iv) in context with comprehensive diagnostics. Furthermore, besides technical innovations and probes, emerging clinical indications are highlighted. Key words: molecular imaging, diagnostic probe, radiotracer, radiomics, multiparametric imaging Running title: Quo Vadis Molecular Imaging? 2 Introduction MI probes and methodologies are often complex in structure and due to their high specificity usually disease-specific, which, in turn, provides advanced differential diagnosis. Furthermore, there is a trend towards multimodal and multiplexed imaging, which requires dedicated readout and image analysis tools, ideally supported by radiomic analysis or artificial intelligence to yield the comprehensive diagnostic power of MI. The pros and cons of the various MI innovations have to be considered not only in the context of the disease but also in liaise whether ultimately a sole diagnostic, theranostic or image guided therapy is needed. The latter will be of specific interest for upcoming cell-based therapies, which are on the horizon, such as CAR T cells or adoptive T cell transfers and provide major opportunities for MI by tailoring therapies according to temporal imaging results. As a matter of fact, in the context of extremely expensive cellular, immune or personalized therapies, costs for imaging will be negligible and ultimately help to reduce costs by optimizing patient stratification and provide precise diagnostic guidance for personalized therapy. However, while the imaging-based guidance of cellular therapies could be straight forward, in context of using imaging for theranostics, there are often discrepancies in dose and toxicity between imaging and therapeutic application of drugs, which have to be considered. The fact that imaging delivers information about pharmacokinetics while a therapeutic drug has to be evaluated in the context of pharmacodynamics, toxicity and its activity profile exhibits often a big gap in the realm of theranostics. Molecular imaging without probes MI with imaging agents provides specific insights into molecular mechanisms, however, for some applications diagnostic findings can also be obtained without specific contrast agents. Naturally, this excludes nuclear medicine approaches since they cannot sufficiently detect natural isotopes. Though, there are modalities capable of providing important endogenous information: optoacoustics, certain optical imaging approaches, and magnetic resonance imaging (MRI). These modalities are able to provide novel tissue-structural or physiologic-functional information without external probes that usually cannot be visualized otherwise. 3 Ultrasound has already come of ages with the emergence of 4D ultrafast supra- resolution ultrasound, demonstrating unsurpassed anatomical resolution. However, another physical phenomenon has recently opened up ultrasound to true molecular imaging without probes: the optoacoustic (or photoacoustic) effect, where pulsed light leads to thermoelastic expansion of chromophores, thus inducing ultrasound signals. There are several intrinsic chromophores in tissues such as hemoglobin contained within blood vessels. Since oxygenated and deoxygenated hemoglobin can be distinguished the method provides information about tissue oxygenation (1). Another excellent intrinsic absorber is melanin, which has led to clinical application in melanoma surgery to detect positive sentinel lymph nodes in patients (2). While these studies have been accomplished with lower resolution scanners, recently a high resolution optoacoustic scanner has been developed and used to characterize severity of psoriasis in patients (3) and in preclinical models response to antivascular therapy (Fig 1A) (4). Furthermore, a label-free metabolic imaging method using mid-infrared optoacoustic microscopy was recently introduced enabling spatiotemporal monitoring of carbohydrates, lipids, and proteins in cells and tissues (Fig 1B). (5) Other label-free methods to image endogenous molecules are provided by MRI, e.g. to assess oxygenation and perfusion of tissues by blood oxygenation level-dependent imaging. Furthermore, endogenous molecules can be assessed using Chemical Exchange Saturation Transfer (CEST) (Fig. 1D)(6)). CEST is based on the transfer of saturated magnetization to the ‘‘bulk’’ water signal. In systems with different pools of protons that can be selectively saturated using different radiofrequencies (RF), transfer of saturated protons from one pool to the other leads to changes in the signal. E.g., glucoCEST can be used to detect endogenous glucose concentration in tumors (7). CEST has already found several clinical applications, e.g. in neurological diseases and CNS tumors (Fig. 1C) (8) but also osteoarthritis (9). Besides this, there is a renaissance of MR spectroscopy, e.g. to better characterize human gliomas by metabolic features (10,11) (Fig. 1C). Molecular tissue characteristics can also be obtained with CT. Recent developments include dual-energy scanners with two X-ray sources exhibiting different spectral characteristics to differentiate between calcium, iodine, and soft tissue via different 4 absorption characteristics of the different materials, e.g. to distinguish small intracranial bleeds from calcium deposits (12). Alternatively, newer technologies enable energy discrimination of the X-rays by the detector, expanding the application into discriminate multiple materials, opening avenues for multiparametric imaging (13). Radiotracers and small molecules Nuclear imaging, specifically positron emission tomography (PET), exhibits not only the advantage of high detection sensitivity compared to other imaging systems but also offers an exceptional flexibility in the design of radiolabeled probes. While for many years imaging tracers based on small molecules and peptides, such as 18F-FDG to quantify glucose metabolism, 68Ga-PSMA for prostate imaging or 68Ga-DOTATATE for imaging somatostatin receptors, were predominantly used for biomedical research and clinical diagnosis (14-16), latest developments toward radiolabeled biologicals, such as antibodies, minibodies, nanobodies or therapeutic cells moved into the focus. Specifically, the emerging field of immune imaging may open unimagined potentials in the realm of diagnosis but also for imaging-based therapy guidance in oncology, autoimmune diseases or neurodegeneration. The outcome of a current clinical study using a 89Zr labeled minibody, specific for CD8 lymphocytes, is eagerly awaited, and will potentially show if immune imaging has the potential to be a paradigm shifter in cancer diagnosis and immunotherapy guidance (17). Other examples are studies with radiolabeled nanobodies to image HER2 expression in breast cancer or macrophages (18,19) or biologicals for imaging PD1 or PD-L1 expression (20). The new area of “imaging biologicals” poses also challenges, and many important details to enable a broad clinical application are not understood yet. Latest technologies offer large flexibilities in the design of biologicals but have to compromise
Details
-
File Typepdf
-
Upload Time-
-
Content LanguagesEnglish
-
Upload UserAnonymous/Not logged-in
-
File Pages20 Page
-
File Size-