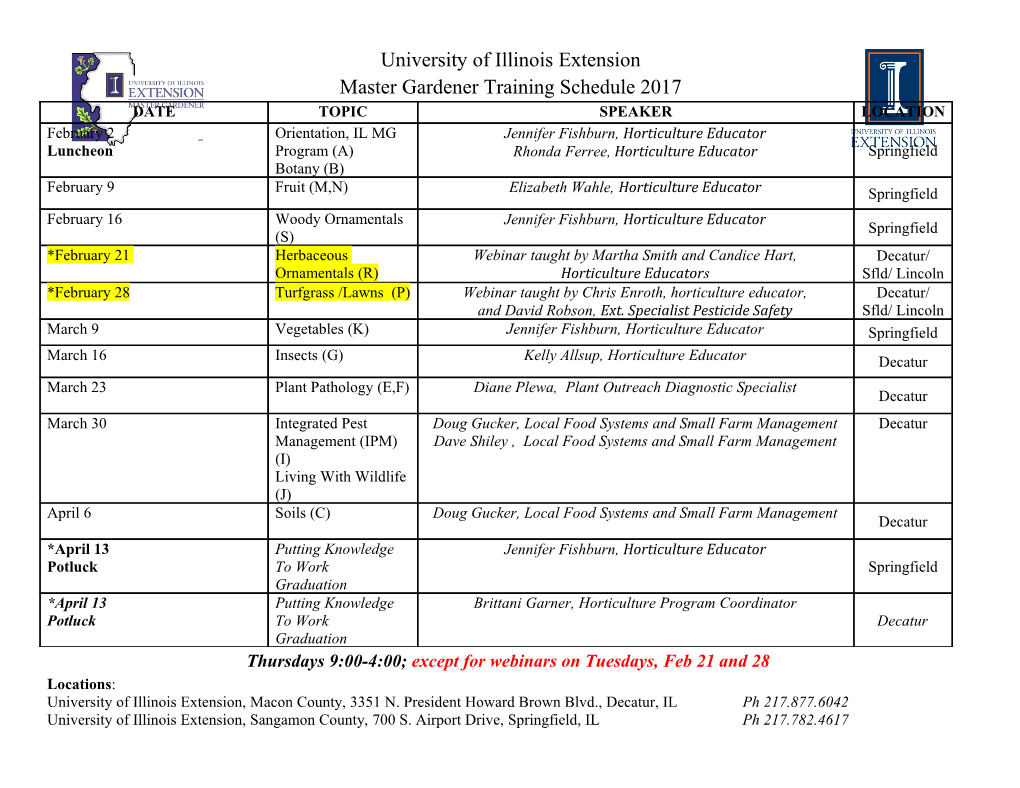
Magnetism as seen with X Rays Elke Arenholz Lawrence Berkeley National Laboratory and Department of Material Science and Engineering, UC Berkeley 1 What to expect: + Magnetic Materials Today + Magnetic Materials Characterization Wish List + Soft X-ray Absorption Spectroscopy – Basic concept and examples + X-ray magnetic circular dichroism (XMCD) - Basic concepts - Applications and examples - Dynamics: X-Ray Ferromagnetic Resonance (XFMR) + X-Ray Linear Dichroism and X-ray Magnetic Linear Dichroism (XLD and XMLD) + Magnetic Imaging using soft X-rays + Ultrafast dynamics 2 Magnetic Materials Today Magnetic materials for energy applications Magnetic nanoparticles for biomedical and environmental applications Magnetic thin films for information storage and processing 3 Permanent and Hard Magnetic Materials Controlling grain and domain structure on the micro- and nanoscale Engineering magnetic anisotropy on the atomic scale 4 Magnetic Nanoparticles Optimizing magnetic nanoparticles for biomedical Tailoring magnetic applications nanoparticles for environmental applications 5 Magnetic Thin Films Magnetic domain structure on the nanometer scale Magnetic coupling at interfaces Unique Ultrafast magnetic magnetization phases at reversal interfaces dynamics GMR Read Head Sensor 6 Magnetic Materials Characterization Wish List + Sensitivity to ferromagnetic and antiferromagnetic order + Element specificity = distinguishing Fe, Co, Ni, … + Sensitivity to oxidation state = distinguishing Fe2+, Fe3+, … + Sensitivity to site symmetry, e.g. tetrahedral, Td; octahedral, Oh + Nanometer spatial resolution + Ultra-fast time resolution Soft X-Ray Spectroscopy and Microscopy 7 Spectroscopy Light/Photon Source Monochromator Sample sample intensity wavelength, photon energy intensity wavelength, photon energy 8 Soft X-Ray Spectroscopy ( h 500-1000eV, 1-2nm) 0 / I t 1.0 0.9 0.8 Electron Storage Ring Monochromator 0.7 Sample 0.6 EY_TM.opj transmitted intensity I intensity transmitted 770 780 790 800 photon energy (eV) 9 X-Ray Absorption Detection Modes 0 / I t 1.0 0.9 0.8 Photons 0.7 absorbed 0.6 EY_TM.opj transmitted intensity I intensity transmitted 770 780 790 800 photon energy (eV) mean 6 free 0 / I path e 5nm 4 Electrons generated 2 electron yield I yield electron 0 EY_TM.opj 770 780 790 800 photon energy (eV) J. Stöhr, H.C. Siegmann, Magnetism (Springer) Electron yield: + Absorbed photons create core holes subsequently filled by Auger electron emission + Auger electrons create low-energy secondary electron cascade through inelastic scattering + Emitted electrons probability of Auger electron creation absorption probability 10 Soft X-Ray Absorption – Probing Depth Element 10eV below L3 at L3 40 eV above L3 1/ [nm] 1/ [nm] 1/ [nm] Fe 550 17 85 Co 550 17 85 Ni 625 24 85 // // // 3nm Mn Fe Co Ni Ru 1.0 NiFe 15nm 0 3nm / I t Ru I 0.8 CoFe 15nm MnIr 15nm // // // luminescence_BL402_Apr2012.opj 640 660 700 720 780 800 860 880 5nm photon energy (eV) Ru 10-20 nm layer thick films supported by substrates transparent to soft X rays 11 X-Ray Absorption Detection Modes and Probing Depth Mn Fe Co Ni e 1.0 1.0 1.0 1.0 0 Ru 3nm / I t I 0.8 0.8 0.8 0.8 NiFe 15nm Ru 3nm 1.1 1.0 CoFe 15nm 1.0 1.4 MnIr 15nm 1.0 1.2 0.9 Ru 5nm 1.0 0.9 luminescence_BL402_Apr2012.opj electron yield (arb. units) 640 650 660 710 720 780 790 850 860 870 photon energy (eV) photon energy (eV) photon energy (eV) photon energy (eV) + Electron sample depth: 2-5 nm in Fe, Co, Ni 60% of the electron yield originates form the topmost 2-5 nm 12 X-Ray Absorption Fundamentals Experimental Concept: Monitor reduction in X-ray flux transmitted through sample as function of photon energy E Charge state of absorber Fe2+, Fe3+ Valence Symmetry of lattice site of absorber: Oh, Td states Sensitive to magnetic order Absorption probability: X-ray energy, X-ray polarization, experimental geometry 2p L 3/2 3 Core level Atomic species of absorber Fe, Co, Ni, …. 2p1/2 L2 13 X-Ray Absorption Fundamentals Experimental Concept: Monitor reduction in X-ray flux transmitted through sample as function of photon energy E Charge state of absorber Fe2+, Fe3+ Valence Symmetry of lattice site of absorber: Oh, Td states Sensitive to magnetic order Absorption probability: X-ray energy, X-ray polarization, experimental geometry 2p L 3/2 3 Core level Atomic species of absorber Fe, Co, Ni, …. 2p1/2 L2 14 ‘White Line’ Intensity Fe valence states 2p L 3/2 3 core level 2p1/2 L2 Intensity of L3,2 resonances is proportional to number of d states above the Fermi level, i.e. number of holes in the d band. 15 ‘White Line’ Intensity Fe valence states 2p L 3/2 3 core level 2p1/2 L2 Intensity of L3,2 resonances is proportional to number of d states above the Fermi level, i.e. number of holes in the d band. 16 ‘White Line’ Intensity Co valence states 2p3/2 L3 core level 2p1/2 L2 Intensity of L3,2 resonances is proportional to number of d states above the Fermi level, i.e. number of holes in the d band. 17 ‘White Line’ Intensity Ni valence states core level 2p3/2 L3 2p1/2 L2 Intensity of L3,2 resonances is proportional to number of d states above the Fermi level, i.e. number of holes in the d band. 18 ‘White Line’ Intensity Cu valence states core level 2p3/2 L3 2p1/2 L2 Intensity of L3,2 resonances is proportional to number of d states above the Fermi level, i.e. number of holes in the d band. 19 X-Ray Absorption Fundamentals Experimental Concept: Monitor reduction in X-ray flux transmitted through sample as function of photon energy E Charge state of absorber Fe2+, Fe3+, … Valence Symmetry of lattice site of absorber: Oh, Td states Sensitive to magnetic order Absorption probability: X-ray energy, X-ray polarization, experimental geometry 2p L 3/2 3 Core level Atomic species of absorber Fe, Co, Ni, …. 2p1/2 L2 20 X-ray Absorption – Valence State Influence of the charge state of the absorber N. Telling et al., Appl. Phys. Lett. 95, 163701 (2009) J.-S. Kang et al., Phys. Rev. B 77, 035121 (2008) 21 X-Ray Absorption – Configuration Model Ni2+ in NiO: 2p6 3d8 → 2p5 3d9 Configuration model, e.g. L edge absorption : + Excited from ground/initial state configuration, 2p63d8 to exited/final state configuration, 2p53d9 + Omission of all full subshells (spherical symmetric) + Takes into account correlation effects in the ground state as well as in the excited state + Leads to multiplet effects/structure http://www.anorg.chem.uu.nl/CTM4XAS/ 2S+1L J. Stöhr, H.C. Siegmann, Magnetism (Springer) 22 X-Ray Absorption Fundamentals Experimental Concept: Monitor reduction in X-ray flux transmitted through sample as function of photon energy E Charge state of absorber Fe2+, Fe3+ Valence Symmetry of lattice site of absorber: Oh, Td states Sensitive to magnetic order Absorption probability: X-ray energy, X-ray polarization, experimental geometry 2p L 3/2 3 Core level Atomic species of absorber Fe, Co, Ni, …. 2p1/2 L2 23 4+ Sensitivity To Site Symmetry: Ti L3,2 PbZr Ti O 3d orbitals 0.2 0.8 2 e e t2 t2 XA (arb. units) J. Stöhr, H.C. Siegmann, + Electric dipole transitions: d02p53d1 Magnetism (Springer) PZT_XA.opj 455 460 465 470 + Crystal field splitting 10Dq acting on 3d orbitals: photon energy (eV) Octahedral symmetry: Tetragonal symmetry: e orbitals towards ligands higher energy e orbitals b2 = dxy, e = dyz, dyz 2 2 2 2 t2 orbitals between ligands lower energy t2 orbitals b1 = dx y , a1 = d3z r 24 X-Ray Absorption Lattice Symmetry TiO2 rutile Influence of lattice site symmetry at the absorber Oh anataseTiO2 ray absorption ray - x D2h G. Van der Laan Phys. Rev. B 41, 12366 (1990) 25 X-Ray Absorption Fundamentals Experimental Concept: Monitor reduction in X-ray flux transmitted through sample as function of photon energy E Charge state of absorber Fe2+, Fe3+ Valence Symmetry of lattice site of absorber: Oh, Td states Sensitive to magnetic order Absorption probability: X-ray energy, X-ray polarization, experimental geometry 2p L 3/2 3 Core level Atomic species of absorber Fe, Co, Ni, …. 2p1/2 L2 26 X-Ray Absorption Fundamentals Experimental Concept: Monitor reduction in X-ray flux transmitted through sample as function of photon energy E Charge state of absorber Fe2+, Fe3+ Valence Symmetry of lattice site of absorber: Oh, Td states Sensitive to magnetic order Absorption probability: X-ray energy, X-ray polarization, experimental geometry 2p L 3/2 3 Core level Atomic species of absorber Fe, Co, Ni, …. 2p1/2 L2 27 Stoner Model For Ferromagnetic Metals + Magnetic moments in Fe, Co, Ni spin-down well described by Stoner model: spin-up d-bands containing up and down empty states, “holes” spins shifted relative to each other empty states, “holes” by exchange splitting + Spin-up and spin-down bands filled exchange splitting according to Fermi statistics + Magnetic moment |m| determined by difference in number of electrons in majority and minority bands J. Stöhr, H.C. Siegmann, Magnetism (Springer) 3d shell maj min |m| μB (ne ne ) 28 Origin of X-ray Magnetic Circular Dichroism 2-Step Model for photoexcitation of electrons from 2p3/2, 2p1/2 to 3d states by absorption 3d of circularly polarized X rays: + Transfer of angular momentum of incident circular polarized X rays to excited electrons (angular momentum conservation, selection rules) + Spin polarization of excited electrons opposite for incident X rays with opposite helicity. + Unequal spin-up and spin-down populations in exchange split valence shell acts as detector for spin of excited electrons. 29 Origin of X-ray Magnetic Circular Dichroism + Calculate transition probabilities from filled 2p3/2 and 2p1/2 states to empty states in d-band for circularly polarized X rays using Fermi’s Golden Rule 30 Origin of X-ray Magnetic Circular Dichroism + Wave functions describe electronic and photon states final state |f Energies include electronic and photon energies final state energy f + Calculate transitions probabilities Tif considering photon as time-dependent perturbation, i.e.
Details
-
File Typepdf
-
Upload Time-
-
Content LanguagesEnglish
-
Upload UserAnonymous/Not logged-in
-
File Pages74 Page
-
File Size-