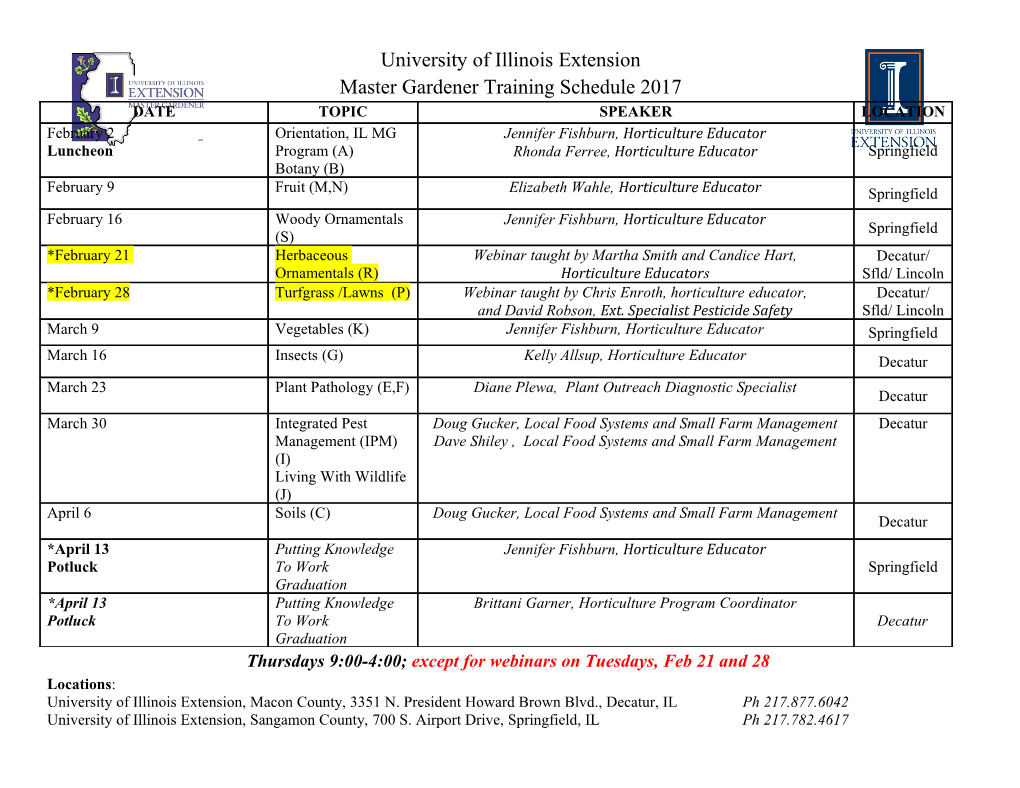
Appendix: methods of studying nucleic acids This appendix describes some of the most ribose or deoxyribose, or purine and py­ important general methods used in the study rimidine. The tissue is treated with cold of nucleic acids not considered elsewhere in dilute trichloroacetic or perchloric acid to this book. The emphasis is on the principles precipitate nucleic acid and protein, and underlying the methods, and no attempt lipid is removed by extraction with organic is made to provide laboratory protocols, solvent. In the procedure of Schneider [ 11] which are now available in abundance, total nucleic acid (DNA + RNA) can be and to which reference will be made. It is separated from protein by hydrolysis in hot inevitable that some of the methods dealt acid, the liberated products being soluble with here will be superseded in the lifetime (section 2.7.3). These latter are then assayed of this edition. The reader wishing inform­ for ribose and deoxyribose. In the procedure ation about current methodologies is there­ of Schmidt and Thannhauser [12] the DNA fore advised to consult the most recent texts. and RNA are separated by alkaline hydro­ However, at the time of writing the cited lysis of the latter followed by acidification references are recommended for more (Fig. A.l). detailed information on principles [ 1-5] and Analysis of nucleic acid phosphorus in practice [6-10]. such fractions usually employs a colour reaction involving the formation of a phos­ phomolybdate complex [13, 14]. Following A.1 OCCURRENCE AND CHEMICAL depurination (section 2.7.2), deoxyribose ANALYSIS can be determined by a colour reaction with diphenylamine (section 2. 7.1) [ 15]. This A.1.1 Chemical determination of nucleic reaction is specific for DNA. In contrast, acids in tissues the orcinol colour reaction employed for depurinated RNA also occurs to a lesser The usual approach to the determination of extent with DNA [16]. The spectral prop­ nucleic acids in tissues involves fractionation erties of the purine and pyrimidine bases of to remove lipids and proteins etc., followed such hydrolysed nucleic acids may also be by assay on the basis of either phosphorus, used as a basis of nucleic acid assay. These 594 Appendix: methods of studying nucleic acids Tissue (section 2.7.4). Protein also absorbs at • t Cold dilu~e TCA or PCA 260 nm, although to a much lesser extent, Organic solven~s dependent on the content of the aromatic amino acids phenylalanine, tryptophan and tyrosine. Because the latter two of these Acid -soluble Nucleic acid + + have an absorption maximum at 280 nm it lipid prorein is possible to obtain an approximate estim­ frachon residue Ho~ TCA or PCA ation of total nucleic acid and protein in Alkaline diges~ion Acidifica~ion a mixture by employing the following formulae based on [17]: Soluble ex~rad Residue Soluble ex~rac~ Residue con~aining conrnining con~aining (pro~ein) Nucleic acid = 0.064 A260 - 0.031 A280 RNA DNA RNA+ DNA (mg/ml) Fig. A. I Extraction and fractionation of nucleic acids from tissues. *TCA - trichloroacetic acid, Protein (mg/ml) = 1.45 A280 - 0.74A260 tPCA- perchloric acid. Typical values for the nucleic acid and bases have absorption maxima in the region protein contents of some rat tissues are of 260 nm (Fig. A.2). The spectral properties given in Table A.l. These may be expressed of double-stranded and single-stranded per gram tissue wet weight, but it is often DNA have already been discussed (section useful to express them in terms of tissue 2.4.1 ), as has the effect of pH thereon protein, which may be easily estimated 16 M 'o l( 14 Ql u c 0 .D L. ~ 6 .D 0 L. 0 4 0 ~ 2 200 320 Fig. A.2 Ultraviolet absorption spectra for purine and pyrimidine bases at pH7. Occurrence and chemical analysis 595 Table A.l Typical values for the concentrations of nucleic acids in different rat tissues Tissue RNA (mglg protein) DNA (mglg protein) RNA/DNA Brain 40 15 2.7 Kidney 43 18 2.4 Liver 54 12 4.5 Skeletal muscle 62 13 4.8 Spleen 58 58 1.0 Thymus 30 93 0.3 Table A.2 Molar percentages of bases in RNAs from various sources Type Adenine Guanine Cytosine Uracil Ribosomal* E. coli 16SrRNA 25.2 31.6 22.9 20.3 23SrRNA 26.2 31.4 22.0 20.4 5SrRNA 19.2 34.2 30.0 16.7 Rat 18S rRNA 22.5 29.2 26.5 21.8 28S rRNA 16.6 35.5 31.6 16.2 5S rRNA 18.3 32.5 27.5 21.7 Messengert E. coli Outer-membrane lipoprotein 28.3 24.2 22.7 24.8 Rabbit P-globin 23.6 27.2 23.0 26.2 Rat a-actin 21.7 26.4 30.6 21.3 Viral* Bacteriophage MS2 23.4 26.0 26.1 24.5 Poliomyelitis virus 29.6 23.0 23.3 24.1 Rous sarcoma virus 23.8 28.8 25.3 22.1 Tobacco mosaic virus 29.1 24.2 19.1 27.6 * Ignoring base modifications. t Ignoring 3'poly(A) tails. [18, 19]. Values of haploid DNA content for scribed for DNA in section 2.2.2. Typical various organisms are given in Table 3.1. values for DNA are given in Table 2.3 and the variation in percentage GC content in different genomes can be found in Table A.1.2 Analysis of base composition and 2.6. The principles of determining the base nearest-neighbour frequency composition of RNA molecules are similar to those for DNA, and values for some The molar proportions of bases in nucleic types of RNA (actually based on sequence acids are determined by hydrolysis, sep­ analysis) are given in Table A.2. Transfer aration and spectral quantification, as de- RNA is not included because of the large 596 Appendix: methods of studying nucleic acids proportion of modified bases (sections 2.1.2 sedimentation rate [25], electron microscopy and 11.7.2). In the case of rRNA (section [26], and radioautography [27]. Generally a 11.6.3) the modified bases have been combination of two or more methods has ignored in Table A.2. It may be remarked been used [28]. that, although it was stated in chapter 12 Although measurement of the sedimen­ that rRNA is GC-rich, this need only be tation rate can be used to estimate the true in relation to the overall base com­ molecular weight of an RNA molecule position of the genome, rather than in (section A.2.2), this method is not readily absolute terms. The examples of mRNAs in applicable to DNA molecules because of Table A.2 are rather arbitrary, and dramatic their extremely large size and asymmetric variations in the base compositions of shape. The theory behind the calculations different mRNAs are seen in the same depends on a knowledge of diffusion co­ orgamsm. efficients but these are equally difficult to The determination of nearest-neighbour obtain either by sedimentation analysis frequencies (i.e. the relative frequency of or optical mixing spectroscopy [29-31]. occurrence of the sixteen different dinu­ However, empirical formulae relating cleotides, NpM) in DNA, although to a sedimentation coefficient (sfo.w) and mole­ considerable extent superseded by nucleo­ cular weight (M) have been derived by tide sequence determination (section A.5), comparing the sedimentation rates of still has applications. It is described in molecules of known size. For example, the section 6.4.3. equation for linear, double-stranded DNA is 0 79 A.1.3 Estimation of the molecular sfo. w = 2.8 + 0.00834 M .4 [28, 32] weight of DNA On sedimentation of DNA to equilibrium Methods of estimating the size of small in density gradients (usually of CsCl - DNA molecules (up to lOkb) using electro­ section A.2.1) the width of the band of phoresis on acrylamide and agarose gels are DNA is inversely proportional to the square described in section A.2.1. Such methods root of the molecular weight. As with light can be accurately calibrated using DNA scattering (see below) the result is depen­ molecules of known sequence. DNA mole­ dent on the concentration of the DNA and cules of up to even 1000 kb in length can be a series of values must be obtained and analysed by pulsed field gel electrophoresis extrapolated to zero concentration [33]. (section A.2.1). This is the most widely used, fundamental Of the more classical approaches, the method of estimating the size of DNA best absolute methods of determining molecules and is applicable to molecules the molecular weight of DNA are light of up to 108 daltons (150 kb ). scattering on low-angle instruments [20-22], Light scattering was widely used in the equilibrium analytical ultracentrifugation in 1950s to measure molecular weights of DNA gradients of caesium chloride [23], and up to 5000 kb. This technique, together with viscoelastic relaxation [24]. Most other conventional analytical velocity centri­ methods have an empirical basis and rely for fugation to determine sedimentation co­ their calibration on the few light-scattering efficients, should lead to reliable absolute experiments that have been performed. determination of molecular weight [34]. But These more empirical methods include the only since the introduction of the laser and measurement of intrinsic viscosity [25] and the production of machines to enable study Occurrence and chemical analysis 597 of scattering at low angles and changes in has been switched off. This viscoelastic the Doppler shift in the wavelength of the effect is caused by the stretched DNA scattered laser light has the method been molecules relaxing to the unstressed con­ extended to molecules of larger size [29-32].
Details
-
File Typepdf
-
Upload Time-
-
Content LanguagesEnglish
-
Upload UserAnonymous/Not logged-in
-
File Pages83 Page
-
File Size-