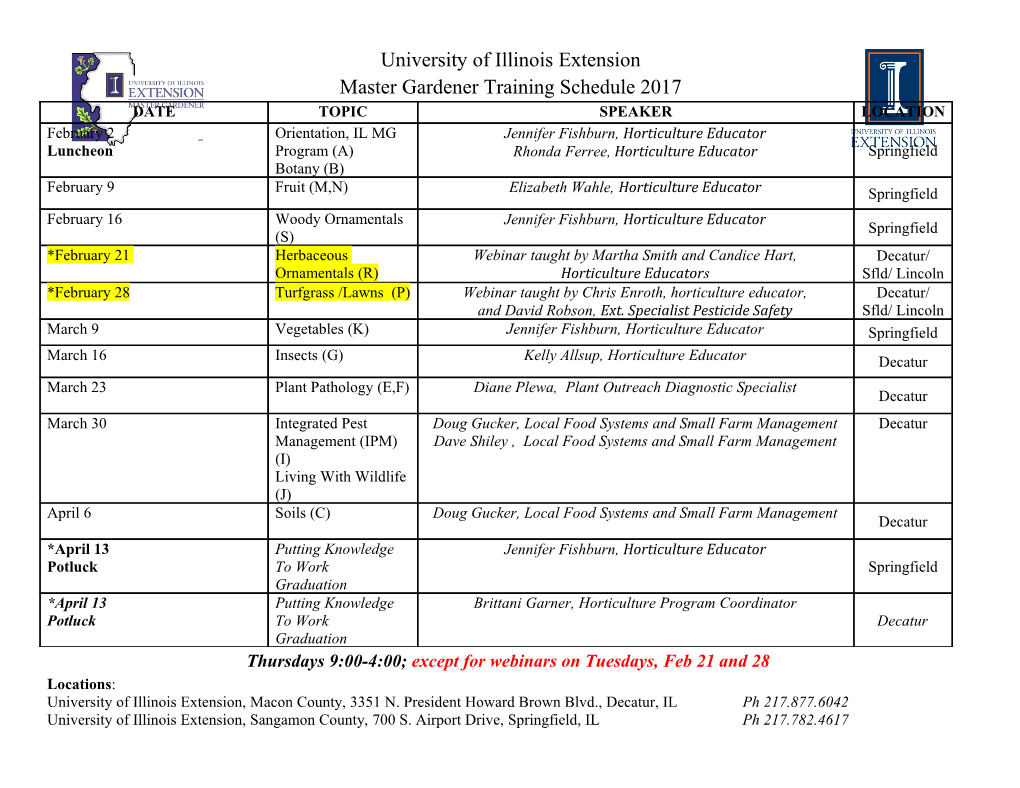
Assessing adaptive genetic variation for conservation and management of the European grayling (Thymallus thymallus) J. V. Huml PhD 2017 Assessing adaptive genetic variation for conservation and management of the European grayling (Thymallus thymallus) Jana Vanessa Huml A thesis submitted in partial fulfilment of the requirements of the Manchester Metropolitan University for the degree of Doctor of Philosophy 2017 Faculty of Science and Engineering Manchester Metropolitan University Abstract In this PhD, functional genetic variation of European grayling (Thymallus thymallus) is assessed to inform conservation and management of the species. This study is the first to characterize immune variation at the Major Histocompatibility complex (MHC) in grayling. The MHC is a marker of high ecological relevance, because of the strong association between immunity and fitness. Taking advantage of advances in sequencing technology, an analytical pipeline optimized for high-throughput, efficient and accurate genotyping of multi-gene families in non-model species is presented. Immune genetic variation is compared to neutral marker data. Results confirm the hypothesis that neutral marker variation does not predict immune genetic variation. Further, the possible effect of supplementing wild populations with hatchery-reared fish on immune genetic variation is evaluated. Significantly lower estimates of heterozygosity were found in stocked than purely native populations. Lower differentiation at immune genes than at neutral markers are indicative of the effects of balancing selection acting upon the MHC, within purely native, but not stocked populations. Furthermore species distribution modelling is used to identify environmental parameters shaping the distribution of grayling. To evaluate risks imposed by climate change, the sensitivity of grayling to climatic variables and range changes under predicted future scenarios are assessed. Locally-optimised mitigation strategies are shown to increase habitat suitability estimates under conditions of climate change. Evolutionary dynamics between hosts and pathogens are important factors in determining an individual’s susceptibility to disease. Studying the microbiome is therefore a promising tool to investigate the risk of disease-mediated extinctions, in relation to environmental conditions and host genetics. This study presents a preliminary analysis on the microbiome of grayling to inform experimental design for future large-scale studies. Altogether, data presented here contribute to improve the management of European grayling. More broadly, it informs conservation research in general, demonstrating the value of taking multiple approaches. Acknowledgements I want to thank the Grayling Research Trust and Manchester Metropolitan University for funding and Plymouth University for hosting me as a visiting student. Also, I thank Dr. Jonathan Ellis for proposing this PhD project. Further, I want to thank the Grayling Society, the Environmental Agency of the UK, the Salmon and Trout Conservation Trust and a number of local angling associations for distributing my request for sampling assistance. I want to thank all the anglers around the UK that have offered their help in sampling: Mike Hodell, Mike Pittendreigh, Munro Reid, Nick Chisholm, Paul Deaville, Pete Turner, Peter Bell, Richard Farrant, Rob Hartley, Roger Smith, Ryan Taylor, Simon Evans, Andrew Cartwright, Bob Male, Chris Backhouse, Christopher Hosker, Dave Hartley, David Baird, David Martin, David Rowley, Dr. N. Pringle, Gareth Evans, Mike Bosomworth, Gary Hyde, Greg Payne, James Munden, Jason Harper, John Goodliffe, John Shillcock, Keith Saunders, Mark Whitmarsh, Martin Bamford, Martin James, Michael Pattinson, Stephen & Helen Wilson, Steve Cheetham, Steve Rhodes, Steve Rimmer, Steve Skuce, Stuart Minnikin, Willie Miller, Robin Mulholland, Tony Barnett, Mark Varey, Alan Swann, David Tibbetts, Karl Jones, Stuart Wardle, Richard Cove, Dave Southall, Tim Jacklin, Ian Hawkins and Jeff Body. Special thanks go to my supervisory team, Dr. Jonathan Ellis, Dr. Ed Harris, Dr. Martin Taylor and Dr. Robin Sen for their guidance, expertise and support. In particular, I want to thank Dr. Jonathan Ellis for his commitment, optimism and patience, which provided me with much support. A lot of helpful inputs were also provided by a number of PhD colleagues and staff both at MMU and Plymouth University, namely I want to thank Dr. Ana Rodiles for introducing me to the QIIME software and Dr. Selvino de Kort for providing me feedback on my progress during annual reviews. Finally, I want to thank my family and friends for giving me confidence. Contents page Chapter 1: Introduction 1 Background 1 The role of genetics in conservation biology 2 Neutral versus functional genetic variation 3 The Major Histocompatibility Complex (MHC) in conservation 5 genetics Metagenomics of microbial communities 7 Species distribution modelling as a tool in conservation biology 8 The European Grayling (Thymallus thymallus) 9 Phylogeography 9 Life history 10 Conservation status and management 11 Study populations 13 Aims and objectives 14 Chapter 2: Novel NGS analysis pipelines for the rapid assessment of highly 17 variable multi-gene families Abstract 17 Introduction 18 Materials and Methods 23 Results 29 Discussion 35 Chapter 3: Evolutionary genetics of European grayling (Thymallus thymallus) 38 Major Histocompatibility (MH) genes Abstract 38 Introduction 39 Materials and Methods 42 Results 44 Discussion 57 Chapter 4: Neutral variation does not predict immunogenetic variation in the 61 European grayling, Thymallus thymallus 61 Abstract Introduction 62 Materials and Methods 65 Results 66 Discussion 77 Chapter 5: The role of climate and other environmental factors in defining 81 habitat suitability of European grayling Abstract 81 Introduction 82 Materials and Methods 85 Results 92 Discussion 99 Chapter 6: Climate change: Risk and mitigation strategies for European 104 grayling Abstract 104 Introduction 105 Materials and Methods 106 Results 107 Discussion 117 Chapter 7: Initial description of the microbiome associated with European grayling 120 Abstract 120 Introduction 121 Materials and Methods 123 Results 125 Discussion 135 Chapter 8: Final discussion 138 Appendices Appendix 1 Analysis scripts 147 Appendix 2 Ewen Watterson test results 156 Appendix 3 Supporting documents of chapter 5 163 Appendix 4 Grayling core microbiome 164 178 References List of Figures page Figure 1.1 Locations of study population 13 Figure 2.1 nested PCR-design for amplicon-library preparation 25 Figure 2.2 Analysis pipeline suggested by Sommer et al. (2013 27 Figure 2.3 Genotyping pipeline 30 Figure 2.4 Relationship between per amplicon coverage and consistency 35 Figure 3.1 Alleles observed per individual for the MH class I α2 45 Figure 3.2 Phylogenetic tree of salmonid MH class I α2 48 Figure 3.3a Alignment of MH class I ZE α1 sequences obtained in this study with the 52 human HLA Figure 3.3b Alignment of MH class II α1 sequences obtained in this study with the 53 human HLA Figure 3.3c Alignment of MH class II β1 sequences obtained in this study with the 54 human HLA Figure 3.4 TREESAAP for MH class I ZE and class II DAA and DAB 55 Figure 4.1 Comparison of genetic diversity at the MH II and microsatellites within native, 70 native stocked and introduced populations Figure 4.2 Relationship between microsatellite and MH II diversity measurements 71 Figure 4.3 Relationship between microsatellite and MH II diversity measurements 72 Figure 4.4 Relationship of pairwise FST /(1- FST ) of microsatellites and MH markers 72 studied Figure 4.5 Population divergence measured as pairwise FST /(1- FST) for microsatellites 73 and MH II markers Figure 4.6: Unrooted phylogenetic trees based on Nei’s genetic distance for 75 microsatellites (from Dawnay et al. 2011), DAA and DAB of the MH class II Figure 4.7 Results of Ewen Watterson tests of neutrality 76 Figure 5.1 Unique occurrence records of European grayling across the entire range 86 spatially rarefied at a scale of 30 km2 Figure 5.2 Unique occurrence records of Thymallus thymallus in the UK spatially rarefied 87 at a scale of 1km2 Figure 5.3 Average predictions of habitat suitability for European grayling across 100 93 replicates Figure 5.4 Maxent estimates of habitat suitability for Thymallus thymallus 95 Figure 5.5 Maxent response curves for all parameters of the final model 97-98 Figure 6.1 Projections of habitat suitability estimates for 2050 for r.c.p. 2.6 (above) and 109 8.5 (below) Figure 6.2 Multivariate environmental similarity surface 110 Figure 6.3 Maximum temperature of the warmest month (Bio5) (°C*10) and mean water 111 temperature (°C) in summer is shown where it exceeds the optimal range for grayling Figure 6.4 Classified predictions of habitat suitability estimates for 2050 for r.c.p. 2.6 and 113 8.5 when locally either orthophoshate, nitrite or copper concentrations were reduced Figure 6.5 Projections of change in habitat suitability estimates for 2050 for r.c.p. 2.6 114 when reducing Orthophosphate, Nitrite or Copper concentrations to maximum values estimated not to affect habitat suitability for grayling Figure 6.6 Projections of change in habitat suitability estimates for 2050 for r.c.p. 8.5 115 when reducing Orthophosphate, Nitrite or Copper concentrations to maximum values estimated not to affect habitat suitability for grayling Figure 6.7 Maximum temperature of the warmest month (Bio5) (°C*10) across the 116 whole area of distribution of European
Details
-
File Typepdf
-
Upload Time-
-
Content LanguagesEnglish
-
Upload UserAnonymous/Not logged-in
-
File Pages216 Page
-
File Size-