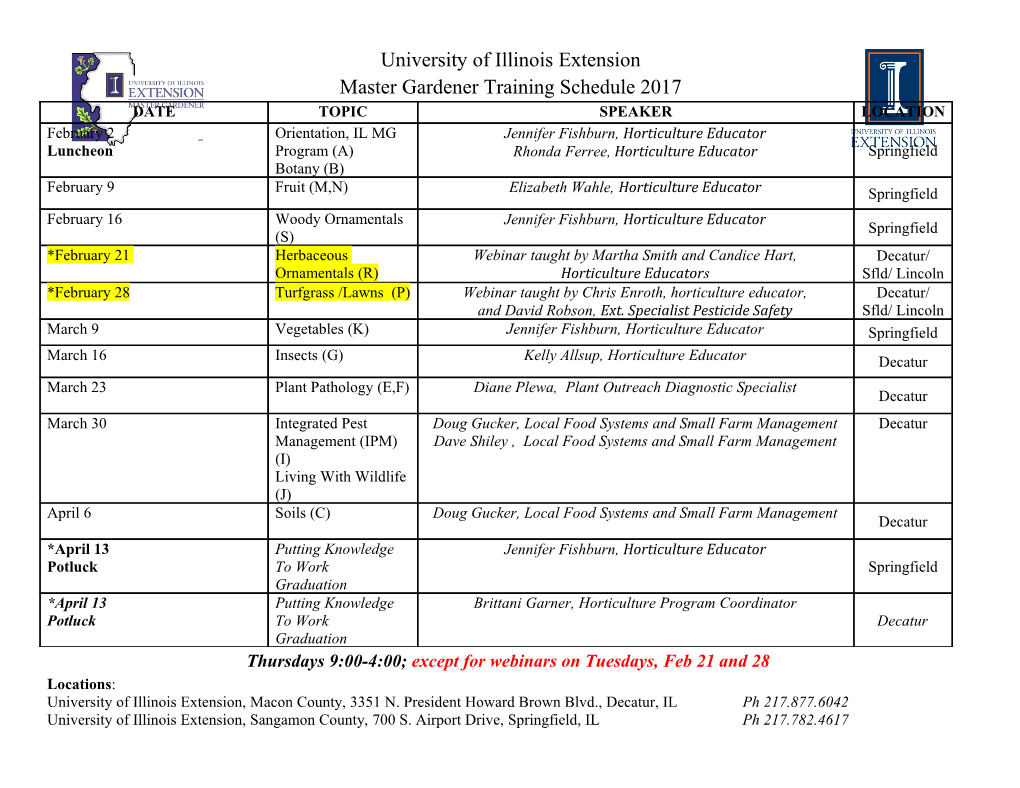
Development and Operation of Miniaturised Ion Traps for Scalable Quantum Computation Dissertation zur Erlangung des Doktorgrades an der Fakultät für Mathematik, Informatik und Physik der Leopold-Franzens-Universität Innsbruck vorgelegt von Felicity Erin Splatt durchgeführt am Institut für Experimentalphysik unter der Leitung von Univ. Prof. Dr. R. Blatt Innsbruck August 2009 Abstract The advent of quantum information processing promises the merging of two of the deepest and most successful scientific and technological developments of the last century: quantum physics and computer science. Ions stored as strings in linear Paul traps are among the most promising systems for the experimental realisation of a quantum device harnessing the computing power inherent in the laws of quantum physics. Scaling ion trap quantum computers to large numbers of qubits requires a new approach. One proposal is the “quantum charge coupled device”, which describes an architecture for a large num- ber of interconnected traps, with the possibility of sorting ions and transporting ions between traps. Implementation of a large number of interconnected traps can be achieved by a segmented(- electrode) ion trap, where the segmentation of the dc electrodes of a linear Paul trap enables a sophisticated control of the axial trapping potential. The promise held by this approach has led to vigourous research in this direction by a number of groups worldwide. The goal of this thesis is the design and construction of a microtrap, and subsequently to gather first experiences in shuttling and sorting mechanisms as required in future quantum compu- tation devices. Work towards this goal has been completed on two fronts: using a rapid-prototype macroscopic printed circuit board trap, and in the design and development of a miniaturised mi- crofabricated segmented ion trap. First experiments were carried out with a segmented surface PCB ion trap. This easy-to- fabricate, rapidly prototyped trap was used to gain a deep understanding of the tailored potentials possible with segmented control electrodes. This knowledge is used in two different ways; in the realisation of long strings of ions, and in the deterministic reordering of ions in a crystal. The macroscopic surface trap has eleven dc electrodes, and the understanding of the effects of these individual electrodes allowed us to realise long (up to 43 ion) strings with almost equal ion spacing. This very long string may be applied to a recent theoretical proposal for quantum computation with ions in an anharmonic trap. In the same trap, ions in a crystal were deterministically reordered – this operation being one of the crucial primitives required of quantum computation in a segmented ion trap. The reordering of ions can be achieved through the use of time-dependent voltages which rotate the principal axes of the trap. These rotations have been implemented in two different ways. In one configuration, a two ion crystal is moved through a path similar to that traversed in a “three-point turn”, the ions finally returning to their origin with positions reversed. This process is deterministic and has a success rate of 93 %. A different path was also realised, a rotation of the weak principal axis around a point, in analogy to a “pirouette”. Here two-, three- and four-ion crystals had their positions reversed, with a success rate of 97 %. This path requires less deviation of the ions from the radiofrequency null and therefore is expected to entail less motional heating. Work was also carried out in the design of a microfabricated segmented electrode ion trap. This two-layer, gold-on-alumina trap has the desirable features of a large number of control electrodes, small dimensions allowing tight confinement and therefore fast gate operations, suppression of axial pseudopotential components, and a design minimising exposed dielectrics. The optimisation of trap dimensions is thoroughly explained, the trap parameters simulated and the fabrication and assembly process is described. iii iv Zusammenfassung Quanteninformationsverarbeitung verspricht die Zusammenführung von zwei der erfolgreichsten Disziplinen von Wissenschaft und Technik, nämlich Quantenphysik und Computerwissenschaften. In linearen Paul-Fallen gespeicherte Ionenketten stellen eine vielversprechende experimentelle Re- alisierung dar, um die Rechengesetze der Quantenmechanik nutzbar zu machen. Bei der Entwicklung eines nützlichen Quantencomputers spielt die Frage der Skalierbarkeit von auf Ionenfallen basierenden Quantencomputern eine zentrale Rolle. Eine mögliche Umsetzung dieses Problems ist eine Fallenarchitektur mit segmentierten Elektroden, welche zahlreiche einzelne Ionenfallen zu einer Gesamtstruktur verbindet und die Möglichkeit zu hochkomplexer Kontrolle des Fallenpotentials bietet. Das Ziel dieser Arbeit ist das Design und die Konstruktion einer Mikrofalle, mit deren Hilfe in Hinblick auf die Entwicklung eines zukünftigen Quantenrechners erste Erfahrungen zu Ionen- transport und -sortierung gesammelt werden können. Die Arbeit behandelt vor allem zwei Aspekte: makroskopische Fallen basierend auf konventioneller Leiterbahn-Technologie zur raschen Erstellung von Prototypen und die Entwicklung von miniaturisierten Mikrofallen. Erste Experimente wurden mit Hilfe einer Oberflächenfalle ausgeführt, welche leicht und vor allem schnell zu realisieren ist. Sie wurde benutzt, um tieferes Verständnis der durch die segmen- tierten Elektroden erzeugten, massgeschneiderten Potentiale zu erlangen. Damit konnten lange Ionenketten realisiert, sowie gezielte Umstellung der Anordnung von Ionen im Ionenkristall gezeigt werden. Diese makroskopische Falle besitzt elf Gleichspannungs-Elektroden, mit deren Hilfe eine Ionen- kette bestehend aus bis zu 43 Ionen mit gleichförmigen Abstand zu den Nachbar-Ionen erzeugt werden konnte. Das könnte eine Anwendung bei der kürzlich (theoretisch) vorgeschlagenen Re- alisierung von Quanteninformationsverarbeitung in einer anharmonischen Falle finden. In der gleichen Falle konnte die Anordnung eines Ionenkristalls gezielt verändert werden - ein Grund- baustein zur Quanteninformationsverarbeitung in einer segmentierten Ionenfalle. Die reproduzier- bare Anordnung kann durch eine zeitabhängige Variation der Fallenpotentiale erreicht werden. Zuerst wurde ein Kristall aus zwei Ionen über einen Pfad mit drei Eckpunkten in die Ausgangspo- sition verschoben, wobei die Positionen der Ionen vertauscht wurden. Ein ähnliches Resultat wurde erzeugt indem das Fallenpotential so verändert wurde, dass sich die Ionen um den gemeinsamen Schwerpunkt drehen. Hier erwartet man weniger Aufheizen der Ionen, da sich die Ionen näher am Radiofrequenz-Minimum befinden. Die Erfolgsrate für den ersten Prozess beträgt 93 %, für den zweiten 97 %. Ein weiterer Teil der Arbeit beschäftigt sich mit der Entwicklung einer mikrostrukturierten segmentierten Ionenfalle. Die Zweischicht-Falle besteht aus einer grossen Zahl von Kontrollelek- troden mit kleiner Ausdehnung und erlaubt starken Einschluss der Teilchen und deswegen schnelle Gatteroperationen. Die Optimierung und Simulation der Fallenparameter, sowie die Fertigung und Zusammenbau der Falle werden beschrieben. v vi Contents 1 Introduction 1 2 Theory 7 2.1 Radiofrequency Ion Traps . 7 2.1.1 Radial Confinement . 8 2.1.2 Axial Confinement: the Linear Paul Trap . 10 2.1.3 The Quantum Charge-Coupled Device: Segmented-Electrode Ion Traps . 11 2.1.4 Linear Paul Trap Geometries with Microfabricated Structures . 12 2.1.5 Ion Strings in a Linear Paul Trap . 12 2.2 Electronic energy Levels of 40Ca+ and 40Ca . 13 2.3 Laser-Ion Interaction . 15 2.3.1 Detection and Measurement . 15 2.3.2 Doppler Cooling . 16 2.3.3 Coherent Manipulations . 17 3 Trap Design and Simulation 19 3.1 Trap Design . 19 3.1.1 Trap Geometry . 20 3.1.1.1 Radial Confinement . 20 3.1.1.2 Axial Confinement . 23 3.1.2 Materials and Fabrication . 28 3.1.2.1 Electrode Material . 29 3.1.2.2 Substrate Material . 30 3.1.2.3 Electrical Breakdown . 31 3.1.3 Practical Considerations . 32 3.1.3.1 Excess Micromotion Compensation . 32 3.1.3.2 Orientation of Trap Axes . 32 3.1.3.3 Ion Loading . 35 3.1.3.4 Trap Packaging and Electrical Connections . 35 3.2 Trap Simulation . 36 3.2.1 Electric Potential Basis Functions ........................ 36 3.2.2 Numerical Simulation Methods . 37 3.2.2.1 The Finite Element Method . 37 3.2.2.2 The Boundary Element Method . 37 3.2.2.3 Comparison between the Boundary Element Method and the Finite Element Method. 38 3.2.2.4 Other Notable Solving Methods . 39 3.2.3 Numerical Field Simulation and Analysis . 42 3.2.3.1 Numerical Field Simulation . 42 3.2.3.2 Electric Potential Analysis . 43 vii Contents 4 Trap Layout and Fabrication 47 4.1 Trap Layout and Simulation of Trap Parameters . 47 4.1.1 Surface trap Bastille ................................ 47 4.1.1.1 Surface Trap Bastille Layout . 48 4.1.1.2 Surface Trap Bastille Numerical Field Simulation and Analysis . 49 4.1.2 Two-Layer Gold-on-Alumina Trap . 61 4.1.2.1 Two-Layer Gold-on-Alumina Trap Geometry . 61 4.1.2.2 Two-Layer Gold-on-Alumina Trap Numerical Field Simulation and Analysis . 63 4.2 Trap Fabrication . 69 4.2.1 Surface Trap Bastille ................................ 69 4.2.2 Two-Layer Gold-on-Alumina Trap . 69 4.2.2.1 Laser Machining of Alumina Wafers . 70 4.2.2.2 Gold Evaporation . 70 4.2.2.3 Electroplating . 71 4.2.2.4 Electrical Insulation . 72 4.2.2.5 Chip Dicing . 72 4.2.2.6 Etching . 73 4.2.2.7 Assembly . 73 5 Experimental Setup 75 5.1 Trap Mounting Hardware and Accessories . 75 5.2 Vacuum
Details
-
File Typepdf
-
Upload Time-
-
Content LanguagesEnglish
-
Upload UserAnonymous/Not logged-in
-
File Pages131 Page
-
File Size-