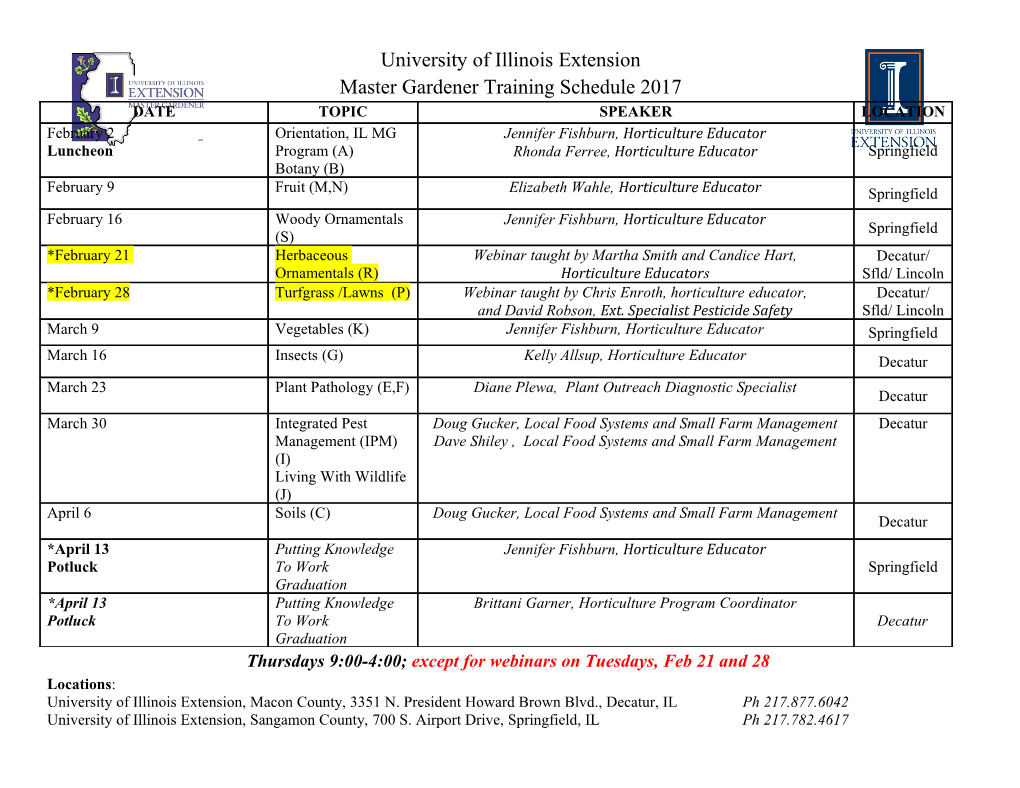
materials Article Enhancement of Bone Ingrowth into a Porous Titanium Structure to Improve Osseointegration of Dental Implants: A Pilot Study in the Canine Model 1, 2, 3 4 5,6 Ji-Youn Hong y , Seok-Yeong Ko y, Wonsik Lee , Yun-Young Chang , Su-Hwan Kim and Jeong-Ho Yun 2,7,* 1 Department of Periodontology, Periodontal-Implant Clinical Research Institute, School of Dentistry, Kyung Hee University, 26, Kyungheedae-ro, Dongdaemun-gu, Seoul 02447, Korea; [email protected] 2 Department of Periodontology, College of Dentistry and Institute of Oral Bioscience, Jeonbuk National University, 567, Baekje-daero, Deokjin-gu, Jeonju-si, Jeollabuk-do 54896, Korea; [email protected] 3 Advanced Process and Materials R&D Group, Korea Institute of Industrial Technology, 7-47 Songdo-dong, Yeonsu-gu, Incheon 406-840, Korea; [email protected] 4 Department of Dentistry, Inha International Medical Center, 424, Gonghang-ro, 84-gil, Unseo-dong, Jung-gu, Incheon 22382, Korea; [email protected] 5 Department of Periodontics, Asan Medical Center, 88, Olympic-ro 43-gil, Songpa-gu, Seoul 05505, Korea; [email protected] 6 Department of Dentistry, University of Ulsan College of Medicine, 88, Olympic-ro 43-gil, Songpa-gu, Seoul 05505, Korea 7 Research Institute of Clinical Medicine of Jeonbuk National University-Biomedical Research Institute of Jeonbuk National University Hospital, 20, Geonjiro, Deokjin-gu, Jeonju-si, Jeollabuk-do 54907, Korea * Correspondence: [email protected]; Tel.: +82-63-250-2289 These authors contributed equally to this study. y Received: 8 May 2020; Accepted: 6 July 2020; Published: 8 July 2020 Abstract: A porous titanium structure was suggested to improve implant stability in the early healing period or in poor bone quality. This study investigated the effect of a porous structure on the osseointegration of dental implants. A total of 28 implants (14 implants in each group) were placed in the posterior mandibles of four beagle dogs at 3 months after extraction. The control group included machined surface implants with an external implant–abutment connection, whereas test group implants had a porous titanium structure added to the apical portion. Resonance frequency analysis (RFA); removal torque values (RTV); and surface topographic and histometric parameters including bone-to-implant contact length and ratio, inter-thread bone area and ratio in total, and the coronal and apical parts of the implants were measured after 4 weeks of healing. RTV showed a significant difference between the groups after 4 weeks of healing (p = 0.032), whereas no difference was observed in RFA. In the test group, surface topography showed bone tissue integrated into the porous structures. In the apical part of the test group, all the histometric parameters exhibited significant increases compared to the control group. Within the limitations of this study, enhanced bone growth into the porous structure was achieved, which consequently improved osseointegration of the implant. Keywords: porosity; dental implant; osseointegration; bone formation; titanium 1. Introduction A dental implant has been accepted as a reliable treatment modality for edentulous ridge with high long-term survival [1], and improvements in implant design, surface treatment, and surgical Materials 2020, 13, 3061; doi:10.3390/ma13143061 www.mdpi.com/journal/materials Materials 2020, 13, 3061 2 of 13 technique led to a marked increase in implant stability [2,3]. However, the results are mostly based on the selection of subjects with the exclusion of any clinical conditions that might have a negative effect on the healing around the implants. There are several possible risk factors associated with early implant failure or impaired healing, including smoking, head and neck radiation [4,5], bone quality and osteoporosis [6]. Osseointegration was defined as a direct and functional connection between bone and an artificial implant. However, the macroscopic (body structure and thread geometry) and microscopic (chemical composition and surface treatment) characteristics of dental implants could influence the success of the osseointegration [7]. The topographical features in an implant surface can be defined in terms of their scales, which were produced by surface modification treatments such as titanium plasma-spraying, grit-blasting, acid-etching, or combinations [2,8]. Apart from the macro-level that is related to the threaded screw of implant geometry and macroporous surface, mechanical interlocking is maximized by microtopographic roughness [9]. In addition, nanotopography is associated with the biological activities of cells to stimulate bone formation on an implant surface [10]. However, the majority of current surface treatments are unreliable to achieve reproducible nanoscale features as they range randomly from nanometers to millimeters. Another approach in surface modification was the production of porous bodies of titanium metal and its alloys, and sintering of metal powders onto the surface was commonly used for porous coatings [11,12]. Advantages of porous surface-enhanced implant include the induction of new bone tissue ingrowth and neovascularization into the porous scaffold in three-dimensional (3D) aspects [13], elastic modulus closer to the cancellous bone that allows load distribution [14], and enhanced transport of metabolites and space for new bone through substantial porous interconnectivity [15]. To maximize the potential benefits from the porous structures, precise control of overall porosity and pore size was identified as important, although the optimal ranges were yet to be determined [16]. However, conventional methods had limitations of low volumetric porosity, irregular dimensions of pores, and poor interconnectivity [17]. Furthermore, possible mechanical failures related to a lack of yield strength and separation of coating materials led to soft tissue encapsulation and loosening of implants [18]. Recent approaches have utilized methods such as selective melting with laser or electron beam, 3D printing, casting or vapor deposition to control the internal pore geometry and distribution [12,19]. The porous scaffolds were sometimes combined with threaded implants for additional advantages in terms of primary mechanical stability and removability. One of the products that had been widely studied to adapt the porous structure to the root form implant was the porous tantalum trabecular metal (PTTM) enhanced implant [18,20,21]. PTTM was fabricated by foam-like vitreous carbon scaffold that resulted in the open-cell structure similar to the trabecular bone [18]. The PTTM part was added to the middle portion of the implant by laser welding and was combined with the screw-type design of titanium alloy surface at the cervical and apical portions, which were microtextured by grit-blasting with hydroxyapatite particles. From the animal studies, histomorphometric evaluations have demonstrated more new bone growth at the PTTM occupying the middle portion compared to the conventional surface within the 12-week study periods, and suggested the potential benefits of the porous structures in the compromised bone quality. However, there were limited biomechanical improvements assessed by the resonance frequency analysis in PTTM and the implant stability appeared comparable to the conventional microtextured surface. In the present study, a novel method of utilizing the powder injection molding technique has been employed to form a porous titanium structure, which was fabricated on the apical portion of the machined screw-type implant. The effect of the newly developed porous structure on osseointegration was compared to the smooth-surfaced implant in the canine model. Materials 2020, 13, 3061 3 of 13 2. Materials and Methods 2.1. Design of the Implants MaterialsA threaded2020, 13, x FOR machined PEER REVIEW surface implant (c.p. titanium grade 4) with an external-type abutment3 of 13 connection (MegaGen Implant Co., Ltd., Gyeongsan, Korea) was used in the control group. The implant measuredimplant body 4.1 mm(core in diameter diameter, of 8.1 3.25 mm mm) in length, with aand homogenous had a straight thread configuration height of 0.35 of the mm implant (Figure body 1a). (coreThe test diameter group ofimplant 3.25 mm) had with a porous a homogenous titanium struct threadure height fabricated of 0.35 on mm the (Figure implant1a). core The at test the groupapical implantportion; hadthe core a porous had titaniuma reduced structure diameter fabricated of 1.25 mm on theand implant a thread core height at the of apical1.35 mm portion; to afford the core the hadspace a reducedfor the porous diameter scaffold of 1.25 (1 mmmm andin depth a thread and height0.83 mm of in 1.35 width) mm to(Figure afford 1b). the The space resulting for the porousprofile scahadff old3-mm (1 mmspiral in shape depth threads and 0.83 in mmthe apical in width) part, (Figure a regular1b). pitch The resultingdistance profileof 1.25 hadmm 3-mmand a thread spiral angle of 45°. shape threads in the apical part, a regular pitch distance of 1.25 mm and a thread angle of 45◦. Figure 1. Design of the implants: (a) overall profiles and dimensions of the implant in the control Figure 1. Design of the implants: (a) overall profiles and dimensions of the implant in the control group; (b) overall profiles and dimensions of the implant in the test group. group; (b) overall profiles and dimensions of the implant in the test group. 2.2. Fabrication of Porous Titanium Structure 2.2. Fabrication of Porous Titanium Structure The porous titanium
Details
-
File Typepdf
-
Upload Time-
-
Content LanguagesEnglish
-
Upload UserAnonymous/Not logged-in
-
File Pages13 Page
-
File Size-