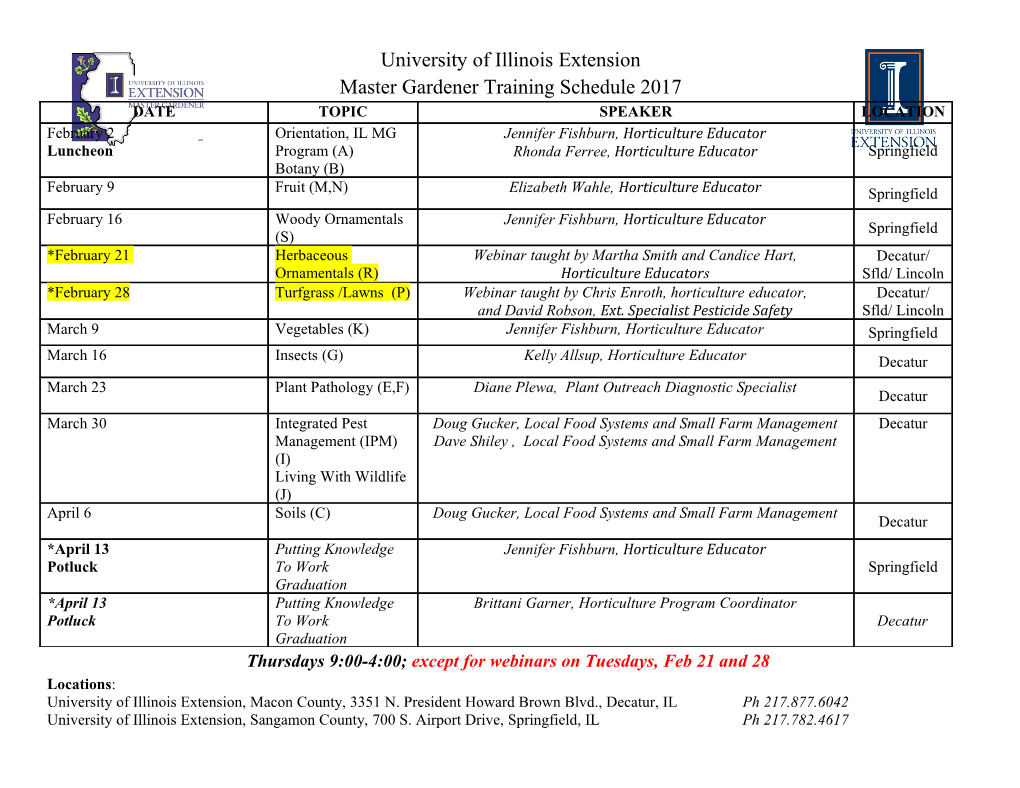
10 Journal of the Meteorological Society of Japan Vol. 59, No. 1 Melting of Snowflakes below Freezing Level in the Atmosphere By Takayo Matsuo and Yoshio Sasyo Meteorological Research Institute, Yatabe-cho, Tsukuba-gun, Ibaraki-ken, 305, Japan (Manuscript received 16 June 1980, in revised form 30 September 1980) Abstract A theoretical and observational approach was made to elucidate the phenomena of melting of snowflakes below freezing level in the atmosphere. A main purpose of the theoretical approach was to examine the effect of relative humidity of air on snowflake melting that has never referred so far. It is based on the consideration that latent heat accompanied with sublimation or condensation of water vapor from or on snowflake surface exerts a significant influence on the melting rate of snowflakes in addition to heat transfer due to heat diffusion from the ambient air to snowflakes. In the theoretical calculations, we used an empirical formula of the melting rate of snowflakes proposed previously by Matsuo and Sasyo (1981) as the basic equation. Snow- flake diameter, liquid water content, and fall velocity as a function of distance below freezing level were obtained by the calculations using parameters such as relative humidity of air, snowflake sizes, and densities. In saturated air below freezing level, snowflakes commenced melting from just below freezing level and snowflakes with equivalent diameter 1-5 mm in raindrops completed melting within several hundred meters. The width of the melting layer thus formed in- creased with increasing sizes and densities of snowflakes contained. In subsaturated air below freezing level, on the other hand, melting of snowflakes did not take place as far as a considerable distance below freezing level because of cooling of snowflakes by sub- limation of water vapor. The width of the non-melting layer thus formed increased nearly linearly as relative humidity decreased; for example, it was about 120 m for relative humidity of 90%, and 700 m for RH=50%. Under the non-melting layer, snowflakes commenced melting because of increase in heat transfer from the ambient air due to increase in air temperature and water vapor density. The width of the melting layer decreased as the layer became dryer. Fall velocity of snowflakes decreased slightly in the non-melting layer and increased rapidly in the melting layer with increasing distance below freezing level. In the observations, simultaneous measurements were carried out for snowflake water content, fall velocity, mass, and cross-sectional area. The observational results showed that fall velocity and liquid water content of snowflakes as a function of their mass were dependent on surface air temperature above 0 * and relative humidity. Especially at high surface air temperature (*1*), fall velocities were almost constant with respect to their masses and some of them in small mass range were higher than those in larger mass range. This finding shows a different tendency from the results observed by Magono (1953) and Langleben (1954). These observational results were interpreted well by the present theoretical calculations. problem of snowflake melting below freezing 1. Introduction level and that there was an intimate relation In the routine weather forecasts, the predic- between the types of precipitation and air tion of types of precipitation on the ground is temperature which precipitation experienced in one of the most important problems. From falling (Sugiyama (1946), Nakazawa and Noto statistical analyses of temperature profiles in the (1971), and Handbook of Weather Forecasting in atmosphere, it was found that the types of the Tokai District. 1974, vol. 1, p. 64). precipitation were closely connected with the In radar meteorology, the problem of melting February 1981 T. Matsuo and Y. Sasyo 11 is also of importance for studies of bright band sectional areas, and water content of snowflakes formation. Most studies of bright band forma- were also measured together with their masses. tion (e.g., Austin and Bemis (1950), Wexler Simultaneous measurements of snowflake fall (1955)) showed that bright band was formed as velocity, cross-sectional area, and mass were a result of various physical processes accom- made in a vertical tunnel which is 50 * 50cm2 panied by snowflake melting. in cross-section and 170 cm in depth. An In spite of the importance of the problem, apparatus used here was described in detail by the study of snowflake melting below freezing Sasyo and Matsuo (1980). The tunnel was level remains at the stage of qualitative inter- darkened inside and set outdoor. Two photo- pretation because of the practical difficulties of couplers, which consist of light emitting diodes the observation and the experiment. A key to and photo-detectors, were placed at different quantitative understanding of the snowflake melt- height with a known interval in the tunnel. The ing is a knowledge of the melting rate of snow- upper photocoupler generated a pulse signal flakes below freezing level. For that purpose, when a falling snowflake shut out light-beam the assessment of physical factors affecting the emmited by diodes and instantly sended it to a melting rate should be made by careful laboratory degital voltmeter equipped with a function of and field studies. The authors, Matsuo and Sasyo time interval measurement, and so did the lower (1981), carried out the melting experiment of one. The degital voltmeter received two signals snowflakes and formulated the melting rate using from two photocouplers for one falling of a physical factors assessed in the experiment. snowflake. The fall velocity could be calculated In this paper, we will make a numerical simu- from a difference in arrival time of two pulse lation of melting of snowflakes below freezing signals. Cross-section of falling snowflakes was level using the empirical formula of the melting photographed from the bottom of the tunnel by rate proposed by the present authors and discuss a motor driven camera with a stroboflash linked the physical factors influencing snowflake melt- electrically with a photocoupler. After the ing below freezing level. In particular, we will snowflake failed on a heated glass plate at the pay attention to the effect of relative humidity bottom of the tunnel, the mass was determined of air which has never described so far. We will by a method of a filter paper dusted with a then support the appropriateness of the simu- water-soluble dye. Cross-sectional areas were lation with a few quantitative observational determined from the cross-sections enlarged on results. photographic papers. Sometimes, snowflake cross- sectional area and fall velocity were missed to be 2. Measurements of fall velocity and liquid measured because of inapposite functioning of the water content of snowflakes apparatus caused by simultaneous falling of two The snowfall observations were carried out in or more snowflakes into the tunnel. January 1978 and February 1979 at Nagaoka, Water content of snowflakes was measured by Niigata prefecture in Japan. Nagaoka is famous a method used by Nakamura (1960). Falling for a snowy country in Japan and is a suitable snowflakes were collected on a filter paper dusted place for studies of snowflake melting because with a water-soluble dye. Liquid water in melt- in the winter monsoon season, Nagaoka is often ing snowflakes was first absorbed by the filter situated in a melting layer. During the periods paper and spotted on it. The trace was out- of the observation, there were about twenty snow- lined by a pencil. Then the ice remaining on the falls and sleet was often found in those snow- paper was melted by warming the paper. The falls. From eye observation, it was found that trace became larger by addition of water pro- most of snowflakes observed in snowfalls con- duced by melting. Liquid water content was sisted mainly of ice crystals of spacial dendrite. deduced from the ratio of the outer trace to the This is suitable in comparing with the observa- inner one. tional results and those present calculated results Autographic records of surface air tempera- which will be obtained using the empirical ture and relative humidity during the observation formula of the melting rate proposed for snow- periods were of use in analysing these data. flakes consisting mainly of ice crystals of spatial dendrite. 3. Numerical simulations of snowflake melting below freezing level Fall velocities of snowflakes were measured simultaneously with their masses and cross- We proposed previously an empirical formula 12 Journal of the Meteorological Society of Japan Vol. 59, No. 1 of the melting rate of snowflakes assuming have any substantial contribution on the melting spherical symmetry of snowflakes (Matsuo and rate of snowflakes. Sasyo (1981)). The rate of decrease in snowflake The assumptions (1) and (2) were used already radius R by melting, which we define as the when the equation (1) was formulated in the melting rate in this paper, could be given by experiment. (3) Temperature of snowflakes remains the freezing point during the melting period. (4) No breaking-up and coalescense of snow- where Lf is latent heat of fusion, Pi initial density flakes do not take place below freezing level. of snowflakes, K thermal conductivity of air, d T (5) Melting is completed when radius of a temperature difference between the environment melting snowflake becomes the equivalent radius and the snowflake, Lv latent heat of vaporization of a raindrop to which the snowflake melts. or condensation of water, an adjustable parame- (6) Lapse rate of air temperature in the ter which was empirically evaluated as 1.75, D atmosphere r is 6*Km-1 and relative humidity molecular diffusion of water vapor in air,* of air RH is constant below freezing level. between water vapor density differenceof the (7) Fall velocity of melting snowflakes is environment and equilibrium water vapor density treated as follows.
Details
-
File Typepdf
-
Upload Time-
-
Content LanguagesEnglish
-
Upload UserAnonymous/Not logged-in
-
File Pages16 Page
-
File Size-