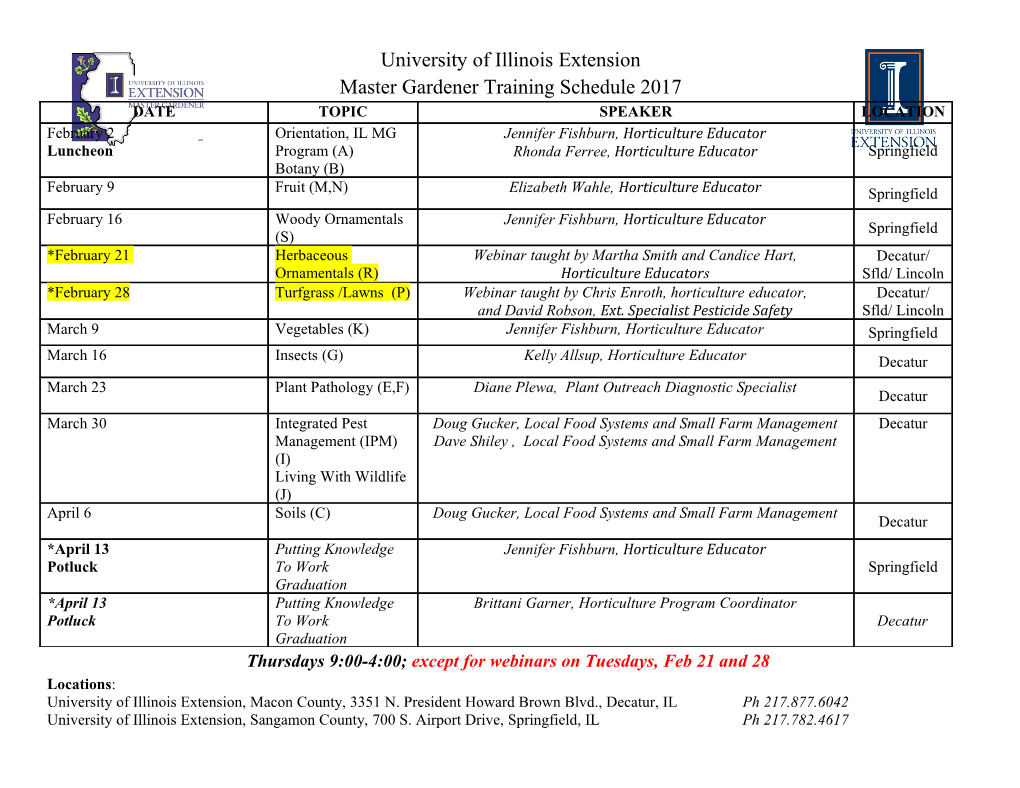
MNRAS 000,1{20 (2017) Preprint 31 October 2017 Compiled using MNRAS LATEX style file v3.0 The Next Generation Transit Survey (NGTS) Peter J. Wheatley,1;2? Richard G. West,1;2 Michael R. Goad,3 James S. Jenkins,4;5 Don L. Pollacco,1;2 Didier Queloz,6 Heike Rauer,7;8 St´ephane Udry,9 Christopher A. Watson,10 Bruno Chazelas,9 Philipp Eigmuller,¨ 7 Gregory Lambert,6 Ludovic Genolet,9 James McCormac,1;2 Simon Walker,1 David J. Armstrong,1;2 Daniel Bayliss,9 Joao Bento,1;11 Fran¸cois Bouchy,9 Matthew R. Burleigh,3 Juan Cabrera,7 Sarah L. Casewell,3 Alexander Chaushev,3 Paul Chote,1 Szil´ard Csizmadia,7 Anders Erikson,7 Francesca Faedi,1 Emma Foxell,1;2 Boris T. G¨ansicke,1;2 Edward Gillen,6 Andrew Grange,3 Maximilian N. Gunther,¨ 6 Simon T. Hodgkin,11 James Jackman,1;2 Andr´es Jord´an,13;14;15 Tom Louden,1;2 Lionel Metrailler,9 Maximiliano Moyano,16 Louise D. Nielsen,9 Hugh P. Osborn,1 Katja Poppenhaeger,10 Roberto Raddi,1 Liam Raynard,3 Alexis M. S. Smith,7 Maritza Soto4, Ruth Titz-Weider7 1Dept. of Physics, University of Warwick, Gibbet Hill Road, Coventry CV4 7AL, UK 2Centre for Exoplanets and Habitability, University of Warwick, Gibbet Hill Road, Coventry CV4 7AL, UK 3Dept. of Physics and Astronomy, University of Leicester, University Road, Leicester, LE1 7RH, UK 4Departamento de Astronomia, Universidad de Chile, Casilla 36-D, Santiago, Chile 5Centro de Astrof´ısica y Tecnolog´ıas Afines (CATA), Casilla 36-D, Santiago, Chile. 6Astrophysics Group, Cavendish Laboratory, J.J. Thomson Avenue, Cambridge CB3 0HE, UK 7Institute of Planetary Research, German Aerospace Center, Rutherfordstrasse 2, 12489 Berlin, Germany 8Center for Astronomy and Astrophysics, TU Berlin, Hardenbergstr. 36, D-10623 Berlin, Germany 9Observatoire Astronomique de l'Universit´ede Gen`eve, 51 Ch. des Maillettes, 1290 Versoix, Switzerland 10Astrophysics Research Centre, School of Mathematics and Physics, Queen's University Belfast, BT7 1NN Belfast, UK 11Research School of Astronomy and Astrophysics, Mount Stromlo Observatory, Australian National University, Cotter Road, Weston, ACT 2611, Australia 12Institute of Astronomy, University of Cambridge, Madingley Rise, Cambridge CB3 0HA, UK 13Instituto de Astrof´ısica, Facultad de F´ısica, Pontificia Universidad Cat´olica de Chile, Av. Vicu~na Mackenna 4860, 7820436 Macul, Santiago, Chile 14Max-Planck-Institut fur¨ Astronomie, K¨onigstuhl 17, 69117 Heidelberg, Germany 15Millennium Institute of Astrophysics, Santiago, Chile 16Instituto de Astronomia, Universidad Cat´olica del Norte, Casa Central, Angamos 0610, Antofagasta, Chile Accepted 2017 October 28. Received 2017 October 27; in original form 2017 September 13 ABSTRACT We describe the Next Generation Transit Survey (NGTS), which is a ground-based project searching for transiting exoplanets orbiting bright stars. NGTS builds on the legacy of previous surveys, most notably WASP, and is designed to achieve higher photometric precision and hence find smaller planets than have previously been de- tected from the ground. It also operates in red light, maximising sensitivity to late K and early M dwarf stars. The survey specifications call for photometric precision of 0.1 per cent in red light over an instantaneous field of view of 100 square degrees, enabling the detection of Neptune-sized exoplanets around Sun-like stars and super- Earths around M dwarfs. The survey is carried out with a purpose-built facility at arXiv:1710.11100v1 [astro-ph.EP] 30 Oct 2017 Cerro Paranal, Chile, which is the premier site of the European Southern Observatory (ESO). An array of twelve 20 cm f/2.8 telescopes fitted with back-illuminated deep- depletion CCD cameras are used to survey fields intensively at intermediate Galactic latitudes. The instrument is also ideally suited to ground-based photometric follow-up of exoplanet candidates from space telescopes such as TESS, Gaia and PLATO. We present observations that combine precise autoguiding and the superb observing con- ditions at Paranal to provide routine photometric precision of 0.1 per cent in 1 hour for stars with I-band magnitudes brighter than 13. We describe the instrument and data analysis methods as well as the status of the survey, which achieved first light in 2015 and began full survey operations in 2016. NGTS data will be made publicly available through the ESO archive. Key words: Atmospheric effects { instrumentation: photometers { techniques: pho- tometric { surveys { planets and satellites: detection { planetary systems © 2017 The Authors ? E-mail: [email protected] 2 P. J. Wheatley et al. Figure 1. The mass-radius relation for known transiting exoplan- ets with masses determined to better than 20 per cent precision (taken from the NASA Exoplanet Archive in January 2017). Plan- Figure 2. The planet and star radii for known transiting exoplan- ets initially discovered in ground-based transit surveys are plotted ets with masses determined to better than 20 per cent precision. as red circles, while those detected from space are plotted as blue Diagonal dotted lines indicate systems with equal transit depth, squares, and planets initially found from radial velocity measure- while the horizontal lines show the radii of solar system plan- ments are plotted as green triangles. Solar system planets are in- ets and the vertical lines are indicative of stellar spectra types dicated with letters and the dotted lines are mass-radius relations (Mamajek, priv. comm., based on Pecaut & Mamajek 2013). The calculated for different compositions by Seager et al.(2007). colours and symbols are the same as Fig.1. 1 INTRODUCTION The photometric detection of transits has proved to be the powerful techniques in exoplanet characterisation is the dis- key to determining a wide range of the physical character- covery of transiting exoplanets, usually in wide-field photo- istics of exoplanets. The depth of a transit depends on the metric surveys. Since most of the characterisation methods relative radii of planet and star (Rp/R∗) and the first transit require high signal-to-noise measurements, there is particu- detections immediately showed that hot Jupiters are gas gi- lar value in the detection of transiting planets around bright ants and not composed primarily of heavy elements (Henry stars. et al. 2000; Charbonneau et al. 2000). Transits also enabled The most successful ground-based surveys for transiting the measurement of stellar obliquities using the Rossiter- exoplanets have been WASP (Pollacco et al. 2006), HAT- McLaughlin effect (Winn et al. 2005), with important im- Net (Bakos et al. 2004) and HATSouth (Bakos et al. 2013), plications for exoplanet migration (e.g. Triaud et al. 2010; which together account for more than 50 per cent of all the Albrecht et al. 2012). They also present the opportunity to known transiting planets with masses determined to bet- determine the composition and structure of planetary at- ter than 20 per cent (including those found from space). mospheres through transmission spectroscopy (e.g. Char- WASP and HATNet employ telephoto lenses mounted on bonneau et al. 2002; Sing et al. 2016), with detections of CCD cameras to make precise photometric measurements Doppler shifts revealing planetary winds (Snellen et al. 2010; over large swaths of the sky, while HATSouth employs 24 Louden & Wheatley 2015) and the detection of deep tran- telescope tubes spread over three locations in the south- sits in ultraviolet lines revealing planetary evaporation (e.g. ern hemisphere. Typically these surveys have found plan- Vidal-Madjar et al. 2003; Ehrenreich et al. 2015). Detec- ets around the mass of Saturn to a few times the mass of tions of secondary eclipses and phase curves in transiting Jupiter, and with radii between that of Saturn and twice systems allow determination of the reflected and thermal Jupiter (Fig.1). A handful of smaller transiting exoplanets emission spectra of exoplanets, together with albedos and have also been found in ground-based transit surveys (Char- the efficiency of heat transport around the planet (e.g. Dem- bonneau et al. 2009; Bakos et al. 2010; Berta-Thompson ing et al. 2005; Charbonneau et al. 2005; Knutson et al. et al. 2015; Gillon et al. 2016, 2017; Dittmann et al. 2017) 2007). When coupled with mass determinations based on the and transits have been found for some planets initially iden- radial-velocities of the star, transits also provide planetary tified in ground-based radial velocity surveys (Gillon et al. densities and hence constraints on their bulk composition 2007; Winn et al. 2011; Bonfils et al. 2012; Dragomir et al. and internal structure (e.g. Seager et al. 2007; Baraffe et al. 2013; Motalebi et al. 2015). The full population of transit- 2008). ing exoplanets with masses determined to better than 20 A prerequisite for the application of this wide range of per cent is shown in Fig.1 (sample taken from the NASA MNRAS 000,1{20 (2017) Next Generation Transit Survey 3 Exoplanet Archive1 in January 2017). The figure illustrates 10 the remarkably diverse nature of the known population, in- Read Dark Sky cluding for instance a factor of eight range in density of Scintillation Jupiter-mass planets. The heating mechanism that inflates Source the low density hot Jupiters remains a matter of debate (e.g. 1 Dark total Spiegel & Burrows 2013). Bright total Space-based surveys, most notably Kepler (Borucki et al. 2010) and CoRoT (Auvergne et al. 2009), have made more precise photometric measurements and have thereby Noise [%] 0.1 discovered transiting exoplanets with smaller radii. These have included rocky exoplanets (e.g. L´eger et al. 2009; Queloz et al. 2009; Batalha et al. 2011), multi-planet sys- tems (e.g. Lissauer et al. 2011) and even circumbinary plan- 0.01 ets (e.g. Doyle et al. 2011; Welsh et al. 2012). Thousands of candidates have been identified, although to date the space- 18 16 14 12 10 8 based surveys have covered a relatively small proportion of I magntiude the sky.
Details
-
File Typepdf
-
Upload Time-
-
Content LanguagesEnglish
-
Upload UserAnonymous/Not logged-in
-
File Pages20 Page
-
File Size-