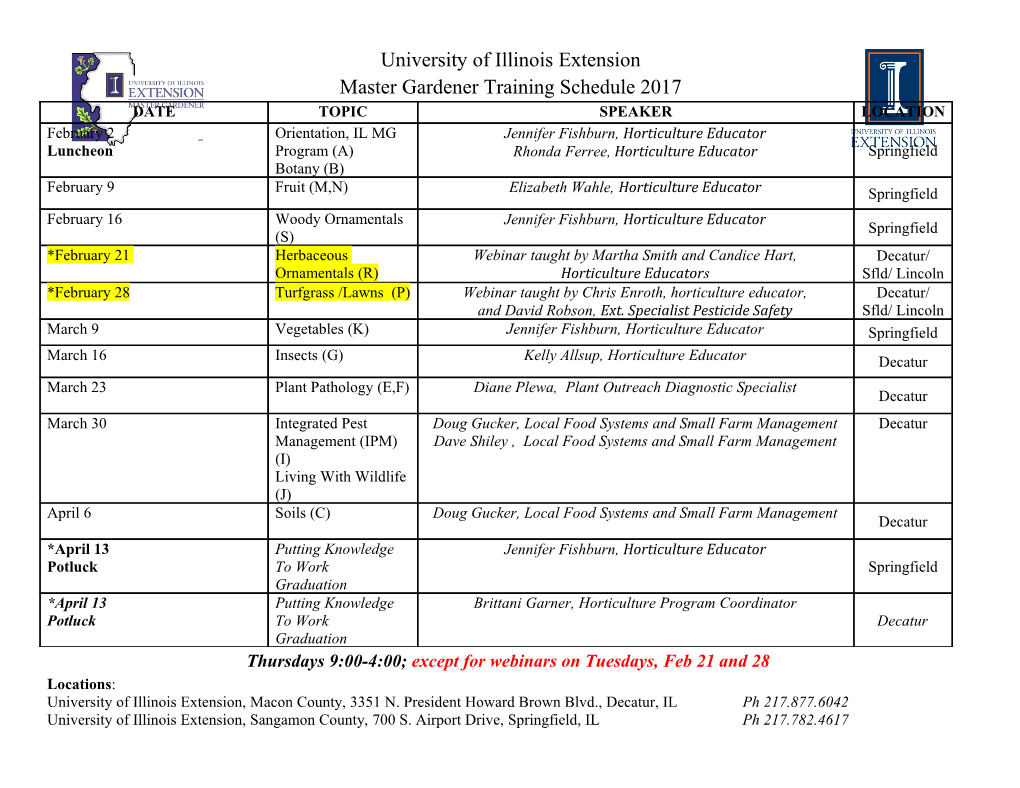
The Journal of Neuroscience, August 15, 1996, 16(16):5225–5232 Haltere Afferents Provide Direct, Electrotonic Input to a Steering Motor Neuron in the Blowfly, Calliphora Amir Fayyazuddin1 and Michael H. Dickinson1,2 1Committee on Neurobiology and 2Department of Organismal Biology and Anatomy, The University of Chicago, Chicago, Illinois 60637 The first basalar muscle (b1) is one of 17 small muscles in flies found one identified haltere campaniform field (dF2) that pro- that control changes in wing stroke kinematics during steering vides strong synaptic input to the mnb1. This haltere to mnb1 maneuvers. The b1 is unique, however, in that it fires a single connection consists of a fast and a slow component. The fast phase-locked spike during each wingbeat cycle. The phase- component is monosynaptic, mediated by an electrical syn- locked firing of the b1’s motor neuron (mnb1) is thought to apse, and thus can follow haltere stimulation at high frequen- result from wingbeat-synchronous mechanosensory input, cies. The slow component is possibly polysynaptic, mediated such as that originating from the campaniform sensilla at the by a chemical synapse, and fatigues at high stimulus frequen- base of the halteres. Halteres are sophisticated equilibrium cies. Thus, the fast monosynaptic electrical pathway between organs of flies that function to detect angular rotations of the haltere afferents and mnb1 may be responsible in part for the body during flight. We have developed a new preparation to phase-locked firing of b1 during flight. determine whether the campaniform sensilla at the base of the halteres are responsible for the phasic activity of b1. Using Key words: haltere; gap junctions; sensory motor reflex; flight; intracellular recording and mechanical stimulation, we have mechanosensory; thoracic ganglion Sensory motor reflexes are conspicuous features of circuits that berg et al., 1986; Hengstenberg, 1988). The control of the wing- maintain the proper orientation of the body during postural stasis stroke resides with a set of 17 small steering muscles that are and locomotion. For example, in the vestibulo-ocular reflex of typically active only during steering maneuvers (Dickinson and vertebrates, information encoding involuntary head movements is Tu, 1996). A notable exception to this rule is the first basalar used to stabilize the visual field on the retina (Carpenter, 1988). muscle (b1), which fires a single action potential at a precise phase An analogous reflex is found among dipterous insects in which the during each wingbeat. During visually induced steering reactions, direction and magnitude of body rotations are encoded and used the firing phase of the b1 may shift 1–2 msec within the wingstroke to make corrective wing and head movements during flight (Faust, (Heide, 1983; Egelhaaf, 1989; Heide and Go¨tz, 1996; Tu and 1952; Sandeman, 1980; Hengstenberg, 1991). However, unlike Dickinson, 1996). These temporal changes, although small, can vertebrates that possess a vestibular system for detecting head affect the biomechanical properties of b1 (Tu and Dickinson, movements, the fly detects changes in body motion with special- 1994) and result in an increased stroke amplitude and adduction ized sensory organs called halteres. of the wing during the downstroke (Tu and Dickinson, 1996). Halteres are barbell-shaped appendages of the third thoracic Although the kinematic consequences of phase shifts in b1 now segment that are derived through evolution from the hind wings. seem clear, the neural mechanisms underlying them are not. During flight, these structures oscillate in antiphase to the wing Wingbeat-synchronous mechanosensory afferents on the wing and and, hence, their massive end knob is subjected to a variety of haltere are thought to provide the feedback for the phase tuning forces. In turn, these forces are encoded into spike trains by of the b1 motor neuron (mnb1) (Heide, 1983; Miyan and Ewing, specialized cuticular strain detectors, campaniform sensilla, that 1984). Haltere afferents are known to terminate in the mesotho- are arranged in five distinct fields at the base of the haltere racic neuropil, where flight motor neurons reside (Chan and (Fraenkel and Pringle, 1938; Pringle, 1948). Although the haltere Dickinson, 1996) and input from a single haltere is sufficient to is subjected to inertial and gravitational forces, the sensory cells provide some phase tuning of both the ipsilateral and contralat- appear most sensitive to Coriolis forces acting on the end-knob eral mnb1s during flight (Heide, 1983). Taken together, these during angular rotation of the body (Nalbach, 1993, 1994). The physiological and anatomical data suggest that haltere afferents compensatory reactions elicited by the halteres include head may provide strong synaptic drive to mnb1. There has been, movements and changes in the wingstroke kinematics (Hengsten- however, no direct demonstration of this sensory motor pathway. In this paper, we will present evidence that the reflex between the Received April 10, 1996; revised May 28, 1996; accepted May 30, 1996. haltere afferents and mnb1 is monosynaptic, that it consists of This study was supported by National Institutes of Health Training Grant T32- both electrical and chemical synapses, and that the electrical GM-07839 to A.F., and by the David and Lucille Packard Fellowship for Science and component appears to be formed by afferents from a single Engineering and National Science Foundation Grant IBN-0208765 awarded to haltere field. M.H.D. We thank Dr. Jonathan Art for use of the piezoelectric crystal used for mechanical stimulation in this study. Correspondence should be addressed to Michael Dickinson, Department of Inte- MATERIALS AND METHODS grative Biology, University of California, Berkeley, CA 94720. Preparation. We used 1- to 3-d-old adult Calliphora vicina obtained from Copyright q 1996 Society for Neuroscience 0270-6474/96/165225-08$05.00/0 a culture maintained in our laboratory. After anesthetizing the flies by 5226 J. Neurosci., August 15, 1996, 16(16):5225–5232 Fayyazuddin and Dickinson • Haltere Input to a Steering Motor Neuron were recorded using an Axoclamp 2A intracellular amplifier (Axon In- struments, Foster City, CA) and filtered at 10 kHz. Extracellular signals from suction electrodes on the b1 nerve and the haltere nerve were amplified with an A-M systems 1800 extracellular amplifier with bandpass filters set at 0.3 and 5 kHz. All signals were digitized using a Vetter 3000A PCM and stored on videotape for later analysis. We used Axotape software (Axon Instruments) for off-line inspection and analysis of the data. Identification of the b1 motor neuron. The mnb1 was located by land- marks and occasionally by visual identification of its primary dendrite. The resting potential of mnb1 ranged from 250 to 260 mV. In prelim- inary experiments, we verified the cell’s identity by iontophoresis of either Lucifer yellow or neurobiotin at the end of an intracellular recording. These fills were then compared with preparations in which the motor neuron had been back-filled from the muscle with biotinylated dextrans and subsequently visualized using diaminobenzidine (DAB) (for proto- col, see Chan and Dickinson, 1996). A camera lucida drawing of a back-filled preparation of mnb1 is shown in Figure 1B. However, ana- tomical verifications of mnb1 recordings were somewhat problematic because of the virtually identical central morphology of mnb1 and mnb2, the motor neuron supplying the second basalar muscle (Fayyazuddin et al., 1993). In cases in which the tracer did not fill the motor neuron axon past the point where it branches from the anterior dorsal mesothoracic nerve (ADMN), it was difficult to distinguish between intracellular fills of mnb1 and mnb2. For these reasons, and to allow the use of lower resistance electrodes, we developed a more rigorous criterion for identi- fying mnb1. In all experiments from which data are presented, we were able to verify intracellular penetrations of mnb1 by recording extracellu- lar spikes from its axon using a suction electrode placed on the b1 nerve, a small branch of the ADMN that supplies b1 (Heide, 1983). The b1 nerve contains only the large axon of mnb1 (;25 mm diameter) (Heide, 1983), a tiny axon from a ventral unpaired median cell supplying the b1 muscle (King and Tanouye, 1983; Tu and Dickinson, 1994), and a few small afferent fibers from sensilla on the side of the thorax (;3 mm diameter) (Heide, 1983). The spikes in the mnb1 axon could be easily distinguished from those of other cells in the b1 nerve because of their large size and their one-for-one relationship with contractions in the b1 muscle. After intracellular penetration, the identity of mnb1 was estab- lished by injecting current to elicit spikes and verifying that extracellularly recorded spikes in the b1 nerve followed the intracellularly recorded spikes with a short latency (,1 msec) and in a one-for-one manner. Bath perfusion. We used a modification of the perfusion method of Hengstenberg (1982) to exchange solutions bathing the thoracic ganglion. Influx was gravity driven through a blunt 30 gauge needle placed just Figure 1. A, Experimental configuration for intracellular recording of anterior to the right wing nerve. The efflux from the preparation flowed mnb1. The preparation was perfused to allow constant exchange of solu- out through a beveled needle placed posterior to the left wing nerve. A tion. Suction electrodes were placed on the b1 nerve to record the activity peristaltic pump (Cole-Parmer) was used to move solution out of the of the mnb1 axon, and on the haltere nerve (HN) to record the response bath. By this method, solutions could be exchanged within 60 sec with of haltere afferents. B, Diagram of Calliphora thoracic ganglion showing minimal movement in the level of the bath solution. The solutions used in location of the mnb1. The arrowhead marks the putative recording site on the various experiments are given in Table 1. the dendrite.
Details
-
File Typepdf
-
Upload Time-
-
Content LanguagesEnglish
-
Upload UserAnonymous/Not logged-in
-
File Pages8 Page
-
File Size-