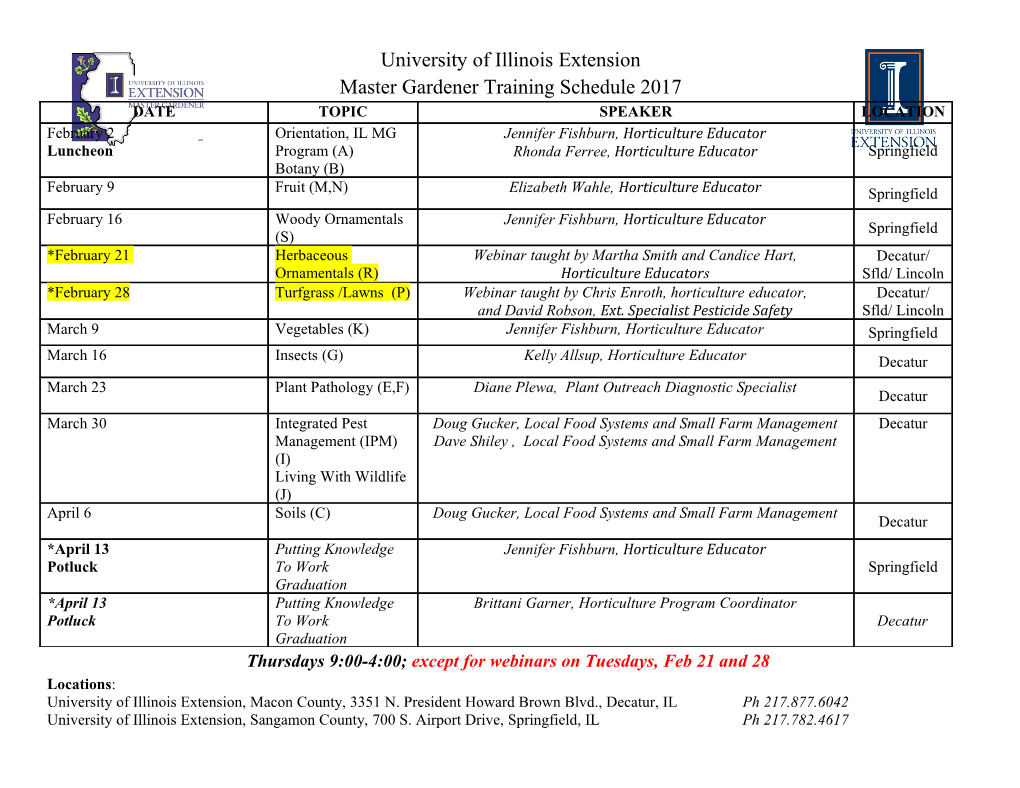
FEATURES Quest for superheavy nuclei 2 P.H. Heenen l and W Nazarewicz -4 IService de Physique Nucleaire Theorique, U.L.B.-C.P.229, B-1050 Brussels, Belgium 2Department ofPhysics, University ofTennessee, Knoxville, Tennessee 37996 3Physics Division, Oak Ridge National Laboratory, Oak Ridge, Tennessee 37831 4Institute ofTheoretical Physics, University ofWarsaw, ul. Ho\.za 69, PL-OO-681 Warsaw, Poland he discovery of new superheavy nuclei has brought much The superheavy elements mark the limit of nuclear mass and T excitement to the atomic and nuclear physics communities. charge; they inhabit the upper right corner of the nuclear land­ Hopes of finding regions of long-lived superheavy nuclei, pre­ scape, but the borderlines of their territory are unknown. The dicted in the early 1960s, have reemerged. Why is this search so stability ofthe superheavy elements has been a longstanding fun­ important and what newknowledge can it bring? damental question in nuclear science. How can they survive the Not every combination ofneutrons and protons makes a sta­ huge electrostatic repulsion? What are their properties? How ble nucleus. Our Earth is home to 81 stable elements, including large is the region of superheavy elements? We do not know yet slightly fewer than 300 stable nuclei. Other nuclei found in all the answers to these questions. This short article presents the nature, although bound to the emission ofprotons and neutrons, current status ofresearch in this field. are radioactive. That is, they eventually capture or emit electrons and positrons, alpha particles, or undergo spontaneous fission. Historical Background Each unstable isotope is characterized by its half-life (T1/2) - the The quest for superheavy nuclei began in the 1940s with the syn­ time it takes for half of the sample to decay. Isotope half-lives thesis of atomic nuclei with a number of protons greater than range from less than a thousandth ofa second to billions ofyears. uranium (Z=92). In 1940, neptunium (Z=93) and plutonium Many radioactive nuclei may have half-lives comparable to or (Z=94) were discovered. This was followed by the synthesis of longer than the age ofthe Earth (4.5 billion years). Examples are americium (Z=95) and curium (Z=96) in 1944, berkelium 1o 8 the actinide nuclei 232Th (TI/2=1·10 years), 23SU (TI/2=7·10 (Z=97) in 1949, californium (Z=98), einsteinium (Z=99) andfer­ 9 years), or 239U (TI/2=4·10 years). Short-lived isotopes cannot be mium (Z=100) in 1952, and mendelevium (Z=lOl) in 1955. All found naturally on Earth because they have long decayed since these elements were produced byintense neutron irradiations or our planet was formed. Yet, thousands ofshort-lived isotopes are byproton, deuteron, or helium (alpha particle) bombardment in continually created in the cosmos. Their existence may be fleet­ a cyclotron. Some ofthese isotopes have been producedinsizable ing, but they play a crucial role in the ongoing formation of the amounts. Einsteinium and fermium were discovered in the elements in the Universe. The properties ofmost rare isotopes are debris from the thermonuclear explosion conducted at Eniwetok unknown and can be only inferred,with considerable uncertain­ Atoll in the Pacific Ocean. Amazingly, the fermium nucleus was ty, from theoretical calculations. made through the capture of 17 neutrons by 23BU followed bythe Figure 1 shows bound nuclear systems as a function of their subsequent betadecays. Innature, a similar process (the so-called proton number Z (vertical axis) and their neutron number N r-process, believed to betaking place in supernova) is responsible (horizontal axis). The black squares represent the stable or very for the synthesis ofheavy elements. long-lived nuclei. They form the so-called "valley of stability". Still heavier elements (transuraniums) were produced in The yellow region indicates shorter-lived nuclei that have been heavy-ion accelerators by fusing heavy actinide targets (plutoni­ produced and studied in laboratories. By adding either protons um-californium) with light ions of carbon (nobelium, Z=102, or neutrons, one moves away from the valley of stability, finally 1958; ruthefordium, Z=104, 1969), boron (lawrencium, Z=103, reaching the drip lines where nuclear binding ends because the 1961), neon (dubnium, Z=105, 1967), and oxygen (seaborgium, forces between neutrons and protons are no longer strong Z=106, 1974). In order to go heavier, to compensate the decrease enough to hold these particles together. of the proton-to-neutron ratio with mass, fusion of nuclei with the largest possible surplus ofneutrons had to be used. Inthe 70s, Nuclear Landscape "cold fusion" reactions involving medium-mass projectiles with Z ~24 and lead or bismuth targets were introduced and replaced "hot fusion" reaction with actinide targets. This enabled the dis­ stable nuclei covery of bohrium (Z=107, 1976), hassium (Z=108, 1984), 82· ~ meitnerium (Z=109, 1982), ununnilium (Z=1l0, 1994), unununium (Z=1l1, 1994), and ununbium (Z=1l2, 1996). The known nuclei .3 - ~-\\ r#. last three elements are still unnamed. So far, they carry tempo­ -t••• rary names derived directly from the atomic number according ~ to the systematic nomenclature of the International Union of Q 50 \ e Pure and Applied Chemistry (IUPAC). 10.. Most ofthe heaviest elements were found in three"heavy ele­ unknown nuclei ment factories": Lawrence Berkeley Laboratory in Berkeley (USA), Joint Institute for Nuclear Research in Dubna (Russia), and Gesellschaft fur Schwerionenforschung (GSI) near Darm­ neutrons Fig. 1: Nuclear landscape stadt (Germany). After much discussion about the priority for 1 8 their synthesis, IUPAC 1997 has accepted names up to Z=109. At europhysics news JANUARY/FEBRUARY 2002 5 Article available at http://www.europhysicsnews.org or http://dx.doi.org/10.1051/epn:2002102 FEATURES .4 I~. ~~- ~ • -r - --'t "- ...-(.=-...... -l ......=--- - - - -- _ = _: that time, nuclei with Z=110, 111, and 112, discovered at GSI, were already known. The lifetimes of the heaviest elements were found to be o Pb, Si - Targets very short. For instance, element if' Pu, Cm - Targets Z=112, investigated using the reac­ U, tion 70Zn + 208Pb ...... 277Uub + In, turned out to have a half life of only Fig. 2: Cross sections for 10-~ about 280 JJsec. The corresponding C the production of the ~ heaviest elements.The fusion cross section is extremely '0-10 small, 1 pb. Since the production ""- results of recent hot fusion experiments with cross section was found to be rapidly I:) '0-11 decreasing with the atomic number, j---------jhot fu ion the 48Ca beam and actinide targets carried see Fig. 2, it was then concluded that :..... t8ea ! out in Dubna are also itwouldbevery difficult to reach still !l . shown in the box. heavier elements. " ._-------', (From Ref. [1] and As far as theory is concerned, Yu.T. Oganessian, private major advances were made in 1966, '0-14 r-'-',---r--,----,-,---r--,-----,-.,--....--,----r-,--..,---,--! communication.) when the first realistic theoretical 102 104 106 108 110 112 114 116 calculations for the superheavy Flement nlJmbp.r nuclei were carried out. These early calculations were' based on the shell correction (or: macroscopic-microscopic) method in which the New discoveries total binding energy was decomposed into a smooth part The last three years (1999-2001) have brought a number of (approximated by a macroscopic liquid drop model) and a experimental surprises which have trulyrejuvenated the field [1]. microscopic quantal shell correction term strongly oscillating InJanuary 1999, scientists from Dubna reported the synthesis of with the number ofnucleons and reflecting the energy stabiliza­ one atom of element 114 <rr:Uuq) in a "hot fusion" reaction tion due to the presence of nucleonic shells. Such calculations between a 48Ca beam and a 244pU target. This discovery was fol­ predicted the nucleus with Z=114, N=184 (mUuq) to be a dou­ lowed by three other reports, also from Dubna. First, using the bly magic center of an island of long-lived superheavy nuclei. 242pU (48Ca,3n) reaction, they produced 287Uuq. In 1999, the syn­ This result stayed practically unchallenged until the late 1990s thesis ofyet another isotope ofZ=114, the even-even 288Uuq, was where more refined models, based on self-consistent mean-field reported. The two a decay sequences were confirmed afterwards theory and realistic effective nucleon-nucleon interactions, start­ (July 2000, May 2001) by the observation ofthree a chains asso­ ed to be systematically applied to superheavy nuclei. In another ciated with the element Z=116 (mUuh) in the 248Cm (48Ca,4o) frontier of superheavy element research, in quantum chemistry, reaction. According to the Dubna analysis, the resulting a-decay relativistic effects in molecules containing heavy elements have chain goes through 288Uuq, 284Uub, and 28OlJun, which subse­ been studied intensively since the mid 1970s, when it became quently fissions.1n neither ofthese cases do the a chains end up apparent that they have to be included in the description ofcom­ at a known isotope so a firm assignment couldnotbe made. Con­ pounds containing heavy elements. sideringthe exponential trend seen inFig. 2 for Z s 114, itactually Chart of the Nuclides 2001 event #5: May 8, 2001 I=-j .....- 291 116 1O.54MeV 4n 55m. /n El 114 ~ -, ..- r 9.80MeV 113113 IM7, );:--I m:ff . , ._---!- :;78 176 ell '12112 . 'U'.N i __ . ! 9.1J M.V "'m 111 :m: : 152.62. 111 111 'Urn. : I lUilP 1H1'" '110 t10 ~10 Ut": OJ'~IIl.j\OO, :1.1 51 Pa, I.l'~ s 1 : ----J ~ :0<,.1 liC '10110 , ,I ~:IIlI'm. mtj ----'IIttW 7~~ 1,'. 172 Fig. 3: The upper end of the nuclear chart in the region of the heaviest elements. The a chain associated with the element mUuh, recently discovered 161 la 1113 , ... (May 8, 2001) in Dubna in the 248Cm (48Ca,4n) reaction, is shown in the upper left corner.
Details
-
File Typepdf
-
Upload Time-
-
Content LanguagesEnglish
-
Upload UserAnonymous/Not logged-in
-
File Pages5 Page
-
File Size-