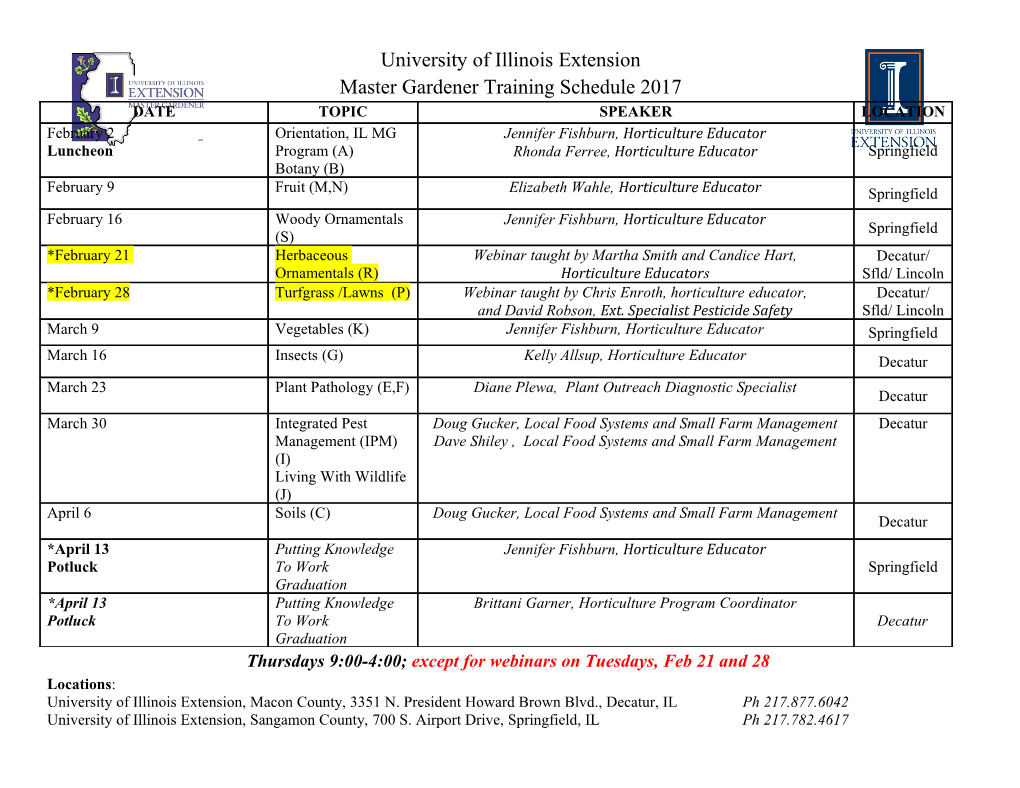
COSTBI-1009; NO. OF PAGES 10 Available online at www.sciencedirect.com Squaring theory with practice in RNA design 1,2 1,3 JP Bida and R Das Ribonucleic acid (RNA) design offers unique opportunities recent work to expand each rule into predictive theories for engineering genetic networks and nanostructures that and tools, highlighting current mismatches between self-assemble within living cells. Recent years have seen the theory and practice. We conclude with a perspective creation of increasingly complex RNA devices, including proof- on trends that may radically accelerate the discovery of of-concept applications for in vivo three-dimensional scaffolding, new RNA design theories and devices. imaging, computing, and control of biological behaviors. Expert intuition and simple design rules — the stability of double helices, Three heuristic design rules the modularity of noncanonical RNA motifs, and geometric All successful RNA designs to date have leveraged three closure — have enabled these successful applications. Going basic rules, many first explored in the interrogation of beyond heuristics, emerging algorithms may enable automated natural RNA systems [3] and the design of DNA devices design of RNAs with nucleotide-level accuracy but, as illustrated [4]. We illustrate these ideas with an ‘RNA square’ on a recent RNA square design, are not yet fully predictive. (Figure 1a–g), recently assembled from eight strands as Looking ahead, technological advances in RNA synthesis and a potential scaffold for nanoscale chemical reactions interrogation are poised to radically accelerate the discovery and (Figure 1a) and crystallized by the Hermann lab [2 ]. stringent testing of design methods. Addresses Rule 1. Watson–Crick base pairs generate stable double helices. 1 Department of Biochemistry, Stanford University, Stanford, CA 94305, Every RNA design made to date has taken advantage of USA 2 helical stems formed as RNA strands double back on Department of Bioengineering, Stanford University, Stanford, CA themselves or associate with other strands to form Wat- 94305, USA 3 son–Crick base pairs. For the RNA square, its edges are four Department of Physics, Stanford University, Stanford, CA 94305, USA helices, each involving association of four strands Corresponding author: Das, R ([email protected]) (Figure 1b). Rule 2. RNA motifs can preserve their behavior when copied and Current Opinion in Structural Biology 2012, 22:xx–yy pasted into new contexts. Explorations of RNAs in living This review comes from a themed issue on Engineering and design systems and elegant in vitro selections have revealed a Edited by Jane Clarke and Bill Schief plethora of natural RNA catalysts, sensors, and structures. Many of these molecules’ functions are due to small (4–15 nucleotide) RNA motifs with non-canonical structure. These motifs can be grafted into if care is taken to avoid 0959-440X/$ – see front matter, Published by Elsevier Ltd. mispairings with flanking sequences. For example, the four http://dx.doi.org/10.1016/j.sbi.2012.06.003 ‘nanocorners’ of the RNA square (Figure 1c) are copies of a five-nucleotide bulge motif, drawn from a right-angle- forming structure in the Hepatitis C virus genome [5]. Ribonucleic acid (RNA) is, in many respects, an ideal polymer for biomolecular design. Natural and designed Rule 3. Geometric closure ensures correct three-dimensional struc- RNA chains can be exquisitely functional: they code for ture. For 3D structures, the geometry of helical stems must genetic information, assemble into intricate three-dimen- successfully interconnect with noncanonical motifs — a sional structures, catalyze chemical reactions, and engage in stringent requirement for RNA designs that encompass nearly every essential biological process in living cells closed ‘ring’ topologies (Figure 1d). For the RNA square, (extensively reviewed in [1]). Most appealingly, these the choice of 10 base pairs for the edges leads to a well- RNA behaviors can be explained and designed by rules ordered square assembly with no detectable alternative that appear strikingly simple and, in favorable cases, are species. Extension to 11 base pairs precludes the closure quantitatively predictive. Indeed, our motivation for study- of the square and gives higher-order assemblies [2 ]. ing RNA design puzzles is that they offer opportunities to discover and rigorously test such predictive rules, which Can these intuitive rules be explained and expanded into might, in turn, enable the modeling of any RNA ‘from quantitatively predictive theories for RNA design? scratch’. Expanding Rule 1: theories for RNA secondary This review focuses on current and missing rules for RNA structure design design, starting with a description of three basic heuristics Physical theories underlying RNA secondary structure in universal use. The three subsequent sections review formation are the most developed models in RNA science www.sciencedirect.com Current Opinion in Structural Biology 2012, 22:1–10 Please cite this article in press as: Bida JP, Das R. Squaring theory with practice in RNA design, Curr Opin Struct Biol (2012), http://dx.doi.org/10.1016/j.sbi.2012.06.003 COSTBI-1009; NO. OF PAGES 10 2 Engineering and design Figure 1 (a) Inner Strand: 5′-CCGGCAGCCU-3 ′ [x4] Outer Strand: 5′-CCGG AGGAACUACUG-3′ [x4] Crystallographic Modeled (b) 5′ (e) C C G A 5′ C C C CGG AG A Pairings with neigh- G G C boring nanocorners G G ′ ′ GU C C G3 3 AU 3′ A U CC G G U C C G C 3′UCCG A A CC G G A G G U 5′CCGG C 5′ A A C (c) (f) Rosetta loop model (5 nucleotides) Sr2+ (d) (g) Cobalt hexammine3+ Mg2+ Rosetta model (outer strand) Current Opinion in Structural Biology A square illustrates RNA design. (a) Sequences (inner and outer) determined ‘by hand’ by Hermann group [2 ]; four copies of the two sequences form the square. (b) Multi-strand secondary structure involves each inner and outer strand forming a nanocorner, and four nanocorners pairing through three-nucleotide sticky ends. (c) Inspiration for the nanocorner, a 5-nt bulge that forms a 90 8C bend in the Hepatitis C virus internal ribosomal entry site (crystallized in PDB ID 2PN4). (d) Crystallographic analysis for full RNA square (PDB ID 3P59). (e) Secondary structure predictions for square design are inaccurate (NUPACK models shown [26]). (f) The ab initio modeling problem of rebuilding the nanocorner’s 5-nt bulge — even given the rest of the coordinates — is not solvable at atomic resolution. (g) An RNA-Puzzle [70 ]: blind prediction of the nanosquare conformation by Rosetta methods is not atomically accurate, even when given the inner strand coordinates as a constraint. and arguably amongst the most predictive theories avail- such as three-way junctions [7–13]. Given such energetic able in biophysics. Several decades of melt experiments models, dynamic programming algorithms implemented on thousands of RNA sequences have been distilled into in packages such as mfold/UNAfold [14], RNAstructure models that parameterize canonical base pair formation [15], and ViennaRNA [16] permit the comprehensive into two dozen ‘nearest-neighbor’ parameters, where the statistical mechanical description of RNA secondary thermodynamic stability of a given base pair depends on structure ensembles for arbitrary sequences (Figure 2b) adjacent base pairs and temperature [6,7]. Further mod- with the ability to recover 70% of phylogenetically eling and measurements have provided approximate determined base pairs [7]. Modeling methods for second- energetic rules (Figure 2a) for many hairpin loops, base ary structure formation kinetics are also under exploration pair mismatches, and more complex inter-helical motifs in packages such as Kinefold [17]. Current Opinion in Structural Biology 2012, 22:1–10 www.sciencedirect.com Please cite this article in press as: Bida JP, Das R. Squaring theory with practice in RNA design, Curr Opin Struct Biol (2012), http://dx.doi.org/10.1016/j.sbi.2012.06.003 COSTBI-1009; NO. OF PAGES 10 Squaring theory with practice in RNA design Bida and Das 3 Figure 2 (a) (b) GUGCA G A A A C +5.7 kcal/mol G Traceback A G A A A G Minimum free G C –2.10 kcal/mol A energy structure or A U –2.40 kcal/mol A A base pair G C probabilities G C U ΔG = +1.2 kcal/mol C (c) Hierarchical Substructure Sequence Global Sequence Target Structure Decomposition Optimization Optimization (d) C U U G C U C U C U G C C U U G U G U G A U G G C G C G C U A G C A A A C G A U A U A A U A A U A C G C G C G C G U A A A A AC GA U A A A U A U A U A U U A U A C G A A U A U A A C G C G C G C G C G C G C G C G C G C G C G A A U A U A U A U A U A C G C G C G A G A G A G 5′ 3′ 5′ 3′ 5′ 3′ 5′ 3′ 5′ 3′ 5′ 3′ -3.0 -4.4 -4.6 -5.2 -5.8 -6.9 kcal/mol No translation Translation Ribosome binding Site Ribosome binding Site 17 °C 37 °C Metastable Hairpins U C U (e) U C AC UC (f) C C U A A U A G U G C G Initiators U AC U A 5′GACCCUAAGCAUACAUCGUCCUUC3′ A U G C C G G C A U G C U A A U A U U A U A H1 C G C G H2 G C G C A U U A A U A U U A U A C G G C C G U A C G A U A U G 3′ G C C mRNA target 5′ U A A G U G G A G A A G A 5′ G Fluorescent in situ U C hybridization chain 3′ reaction H2 H2 H2 H1 H1 Tethered Fluorescent H2 H2 H2 Amplification Polymers H1 H1 H1 H2 H2 H2 H1 H1 H1 Current Opinion in Structural Biology Theory and practice in RNA secondary structure design.
Details
-
File Typepdf
-
Upload Time-
-
Content LanguagesEnglish
-
Upload UserAnonymous/Not logged-in
-
File Pages10 Page
-
File Size-