Effect of Solvent on Gd(DOTA)– Complex Formation: a Preliminary Investigation
Total Page:16
File Type:pdf, Size:1020Kb
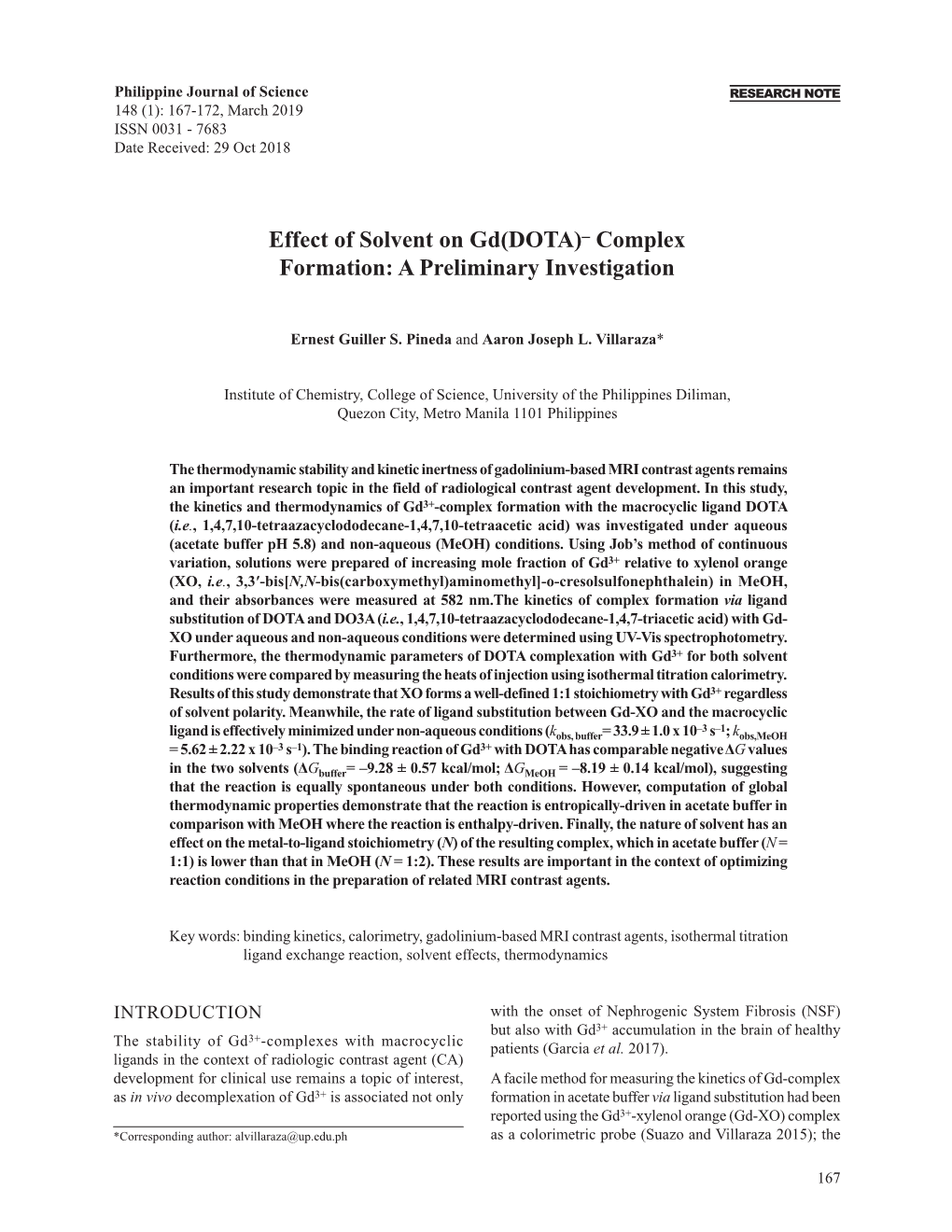
Load more
Recommended publications
-
Spectroscopic Study of the Salicyladazine Derivative–UO2
nanomaterials Article Spectroscopic Study of the Salicyladazine 2+ Derivative–UO2 Complex and Its Immobilization to Mesoporous Silica Sujin Park 1, Jaehyeon Park 1, Ji Ha Lee 2,*, Myong Yong Choi 1,* and Jong Hwa Jung 1,* 1 Department of Chemistry and Research Institute of Natural Sciences, Gyeongsang National University, Jinju 52828, Korea; [email protected] (S.P.); [email protected] (J.P.) 2 Department of Chemistry and Biochemistry, The University of Kitakyushu, Hibikino, Kitakyushu 808-0135, Japan * Correspondence: [email protected] (J.H.L.); [email protected] (M.Y.C.); [email protected] (J.H.J.); Tel.: +82-55-772-1488 (J.H.J.) Received: 2 April 2019; Accepted: 26 April 2019; Published: 2 May 2019 Abstract: Uranyl ion, the most soluble toxic uranium species, is recognized as an important index for monitoring nuclear wastewater quality. The United States Environmental Protection Agency (US EPA) and the World Health Organization (WHO) prescribed 30 ppb as the allowable concentration of uranyl ion in drinking water. This paper reports on a nanohybrid material that can detect uranyl ions spectroscopically and act as a uranyl ion absorbent in an aqueous system. Compound 1, possessing a salicyladazine core and four acetic acid groups, was synthesized and the spectroscopic properties of 2+ its UO2 complex were studied. Compound 1 had a strong blue emission when irradiated with UV 2+ 2+ light in the absence of UO2 that was quenched in the presence of UO2 . According to the Job’s 2+ plot, Compound 1 formed a 1:2 complex with UO2 . -
Research Article Design and Synthesis of a Fluorescent Probe Based on Copper Complex for Selective Detection of Hydrogen Sulfide
Hindawi Journal of Sensors Volume 2021, Article ID 8822558, 7 pages https://doi.org/10.1155/2021/8822558 Research Article Design and Synthesis of a Fluorescent Probe Based on Copper Complex for Selective Detection of Hydrogen Sulfide Guanglan Mao ,1 Chenxi Liu ,2 Nan Yang ,2 Linlin Yang ,2 and Guangjie He 2 1Department of Traditional Chinese Medicine, The Third Affiliated Hospital of Xinxiang Medical College, East Section of Hualan Avenue, Xinxiang, 453000 Henan Province, China 2Xinxiang Key Laboratory of Forensic Science Evidence, School of Forensic Medicine, Xinxiang Medical University, Jinsui Road No. 601, Xinxiang, 453003 Henan Province, China Correspondence should be addressed to Guangjie He; [email protected] Received 20 September 2020; Revised 29 January 2021; Accepted 2 February 2021; Published 16 February 2021 Academic Editor: Roberto Paolesse Copyright © 2021 Guanglan Mao et al. This is an open access article distributed under the Creative Commons Attribution License, which permits unrestricted use, distribution, and reproduction in any medium, provided the original work is properly cited. fl 2+ A novel uorescence probe NA-LCX was rationally designed and synthesized for the sequential recognition of Cu and H2S by the 2+ combination of hydroxyl-naphthalene and diformylphenol groups. The response properties of NA-LCX for Cu ions and H2S with “on-off-on” manner were investigated by fluorescence emission spectra. A highly selective and sensitive response of complex NA- 2+ μ LCX-Cu for H2S over other competing amino acids was observed with a limit of detection at 2.79 M. The stoichiometry of NA- LCX toward Cu2+ ions was determined to be 1 : 1 by the UV-Vis absorption spectrum, and the coordination configuration was calculated by density functional theory (DFT) calculations. -
Dual Sensing Performance of 1,2-Squaraine for the Colorimetric Detection of Fe3+ and Hg2+ Ions
materials Article Dual Sensing Performance of 1,2-Squaraine for the Colorimetric Detection of Fe3+ and Hg2+ Ions Xiaoqian Liu 1,* , Na Li 1, Min-Min Xu 2 , Chunhui Jiang 3, Jianhao Wang 1, Guoqiang Song 1,* and Yong Wang 2,* 1 School of Pharmaceutical Engineering and Life Science, Changzhou University, Changzhou 213164, China; [email protected] (N.L.); [email protected] (J.W.) 2 College of Chemistry, Chemical Engineering and Materials Science, Soochow University, Suzhou 215123, China; [email protected] 3 School of Environmental and Chemical Engineering, Jiangsu University of Science and Technology, 2 Mengxi Road, Zhenjiang 212003, China; [email protected] * Correspondence: [email protected] (X.L.); [email protected] (G.S.); [email protected] (Y.W.) Received: 12 September 2018; Accepted: 12 October 2018; Published: 16 October 2018 Abstract: A simple 1,2-squaraine based chemosensor material (SQ) has been reported to show dual sensing performance for colorimetric detection of Fe3+ and Hg2+ ions. Compared to common instrumental analysis, this method could provide fast and direct detection though colorimetric changes by the naked eye. The sensor has shown excellent selectivity over the other metal ions by tuning different solvent environments. The detection limit for Fe3+ could reach to 0.538 µM, which was lower than that in the environmental agency guideline (U.S. Environmental Protection Agency, U.S. EPA) in drinking water. And for Hg2+ detection, the limit was calculated as 1.689 µM in our case. A 1:1 binding mode between SQ–Fe3+ and SQ–Hg2+ ion were evidenced by Job’s plot measurement and IR analysis. -
Spectrophotometric Detection of Glyphosate in Water by Complex Formation Between Bis 5-Phenyldipyrrinate of Nickel (II) and Glyphosate
water Article Spectrophotometric Detection of Glyphosate in Water by Complex Formation between Bis 5-Phenyldipyrrinate of Nickel (II) and Glyphosate Aline Romero-Natale 1, Ilaria Palchetti 2 , Mayra Avelar 3,4, Enrique González-Vergara 1 , José Luis Garate-Morales 5 and Eduardo Torres 1,* 1 Centro de Química, ICUAP, Benemérita Universidad Autónoma de Puebla, 14 Sur y Av. San Claudio, Col. San Manuel, Puebla 72570, Mexico; [email protected] (A.R.-N.); [email protected] (E.G.-V.) 2 Dipartimento di Chimica, Università Degli Studi di Firenze, Via della Lastruccia 3, 50019 Sesto Fiorentino (Fi), Italy; ilaria.palchetti@unifi.it 3 Departamento de Ingeniería Celular y Biocatálisis, Instituto de Biotecnología Universidad Nacional Autónoma de México, Av. Universidad 2001 Chamilpa, Cuernavaca 62210, Morelos, Mexico; [email protected] 4 Department of Biotechnology, Chemistry, and Pharmacy, University of Siena, Via A. Moro 2, 53100 Siena, Italy 5 Facultad de Ciencias Químicas, Benemérita Universidad Autónoma de Puebla, 14 Sur y Av. San Claudio, Col. San Manuel, Puebla 72570, Mexico; [email protected] * Correspondence: [email protected]; Tel.: +52-222-2295500 (ext. 7273) Received: 26 February 2019; Accepted: 3 April 2019; Published: 6 April 2019 Abstract: A spectrophotometric method for the determination of glyphosate based on the monitoring of a complex formation between bis 5-phenyldipyrrinate of nickel (II) and the herbicide was developed. The method showed a short response time (10 s), high selectivity (very low interference from other pesticides and salts), and high sensitivity (LOD 2.07 10 7 mol/L, LOQ 9.87 10 7 mol/L, and × − × − a K from 1.75 10 6 to 6.95 10 6 mol/L). -
Supporting Information for Reversible Covalent Locking of A
Electronic Supplementary Material (ESI) for Polymer Chemistry. This journal is © The Royal Society of Chemistry 2020 Supporting information for Reversible covalent locking of a supramolecular hydrogel via UV-controlled anthracene dimerization Zhanyao Hou,a Werner M. Nau,b Richard Hoogenboom*a a. Supramolecular Chemistry Group, Centre of Macromolecular Chemistry (CMaC), Department of Organic and Macromolecular Chemistry, Ghent University, Krijgslaan 281-S4, 9000 Gent, Belgium b. Life sciences and chemistry, Jacobs University Bermen, Campus Ring 1, 28759 Bremen, Germany Experimental section Materials All chemicals and solvents were commercially available and used as received unless otherwise stated. Dichloromethane (DCM), N,N-dimethylacetamide (DMA), THF, methanol, CDCl3, hexane were obtained from Sigma Aldrich. DCM and THF was purified over aluminum oxide by means of a solvent purification system from J.C. Meyer when it was use as reaction solvent. Milli-Q Water (18.2 MΩ/cm) was generated using a Millipore Milli-Q academic water purification system. 2- anthracenecarboxylic acid, N-acryloylmorpholine and γ-cyclodextrin were obtained from Tokyo Chemical Industry (TCI). 2-(2-Aminoethoxy)ethanol (98%), Di-tert-butyldicarbonate (99%), 2- dimethylaminoethanol, 2-bromoethanol, trifluoroacetic acid (TFA, 99%) and pentafluorophenol were purchased from Sigma-Aldrich. Azobisisobutyronitrile (AIBN, 98%, Sigma-Aldrich) was recrystallized from MeOH (2x) and stored in the freezer. Methyl-2-(n- butyltrithiocarbonyl)propanoate (MBTTCP) was prepared according to the established procedures.1 Cucurbit[8]urils (CB[8]) was synthesized according to a literature procedure and was kindly provided by Prof. Werner Nau.2 Analytical techniques 1H and 13C spectra were acquired on a Bruker Avance 400 MHz spectrometer. 19F NMR spectra were recorded on a Bruker Avance 500 MHz spectrometer. -
Synthesis of Various Catalysts for the Morita-Baylis-Hillman Reaction
H-SC Journal of Sciences (2016) Vol. V Lam et al. Synthesis of Various Catalysts for the Morita-Baylis-Hillman Reaction Benjamin W. Lam ‘17, A. Mitchel Owens ‘16, John W. Sheffield ‘16, and Paul H. Mueller Department of Chemistry, Hampden-Sydney College, Hampden-Sydney, VA 23943 INTRODUCTION The Morita Baylis-Hillman reaction is a process that has been related to increasing the enantiomer excess of the molecules synthesized. This reaction is a condensation of an acrylate ester and an aldehyde forming a α-methylene-β-hydroxy ester as seen in Figure 1. This reaction as stated above is particularly useful to synthetic chemists for its ability to synthesize chiral molecules in a surrounding involving a chiral catalyst. This chiral catalyst generally is a tertiary amine or phosphine based on known literature. These catalysts act as a ligand binding on to the specific aldehyde and 1 Figure 2. Scheme for the Morita Baylis-Hillman reaction acrylate ester using its bifunctional properties. utilizing a bifunctional metal ligand complex as a catalyst O OH O O Nucleophile (Nu) + R H Me R Me Aldehyde Acrylate Ester α-methylene-β-hydroxyacid ester Figure 1. The Morita Baylis-Hillman reaction involving the condensation of an aldehyde with an acrylate ester to form an α-methylene-β-hydroxy ester. The imine ligand catalyst that would be synthesized would have both a Lewis acid and a Lewis base part to its structure. These parts act as a binding mechanism to form the α-methylene-β- Figure 3. Mechanism for the Morita Baylis-Hillman reaction: hydroxy ester. -
A Benzothiazole-Based Fluorescent Probe for Ratiometric Detection of Al3+ and Its Application in Water Samples and Cell Imaging
International Journal of Molecular Sciences Article A Benzothiazole-Based Fluorescent Probe for Ratiometric Detection of Al3+ and Its Application in Water Samples and Cell Imaging Zhen-Nan Tian, Ding-Qi Wu, Xue-Jiao Sun, Ting-Ting Liu and Zhi-Yong Xing * Department of Applied Chemistry, College of Arts and Sciences, Northeast Agricultural University, Harbin 150030, China; [email protected] (Z.-N.T.); [email protected] (D.-Q.W.); [email protected] (X.-J.S.); [email protected] (T.-T.L.) * Correspondence: [email protected] Received: 29 October 2019; Accepted: 26 November 2019; Published: 28 November 2019 Abstract: An easily prepared benzothiazole-based probe (BHM) was prepared and characterized by general spectra, including 1H NMR, 13C NMR, HRMS, and single-crystal X-ray diffraction. Based on the synergistic mechanism of the inhabitation of intramolecular charge transfer (ICT), the BHM 3+ displayed high selectivity and sensitivity for Al in DMF/H2O(v/v, 1/1) through an obvious blue-shift in the fluorescent spectrum and significant color change detected by the naked eye, respectively. The binding ratio of BHM with Al3+ was 1:1, as determined by the Job plot, and the binding details were investigated using FT-IR, 1H NMR titration, and ESI-MS analysis. Furthermore, the BHM was successfully applied in the detection of Al3+ in the Songhua River and on a test stripe. Fluorescence imaging experiments confirmed that the BHM could be used to monitor Al3+ in human stromal cells (HSC). Keywords: benzothiazole; ratiometric; fluorescent probe; Al3+ 1. Introduction Aluminum, as one of the most abundant metals in the earth, is widely used in daily life, including in kitchen utensils, pharmaceutical packaging, and food additives [1–4]. -
A Coumarin-Based Sensitive and Selective Fluorescent Sensor for Copper(II) Ions† Cite This: New J
NJC View Article Online PAPER View Journal | View Issue A coumarin-based sensitive and selective fluorescent sensor for copper(II) ions† Cite this: New J. Chem., 2014, 38, 4434 Jiun-Ting Yeh, Wei-Chieh Chen, Shi-Rong Liu and Shu-Pao Wu* A new coumarin-derived fluorescent probe (1) exhibited significant fluorescence quenching in the presence of Cu2+ ions. Other metal ions, e.g. Ag+,Ca2+,Cd2+,Co2+,Fe2+,Fe3+,Hg2+,K+,Mg2+,Mn2+, Ni2+,Pb2+, and Zn2+, produced only minor changes in the fluorescence of chemosensor 1. The binding Received (in Montpellier, France) 2+ 30th April 2014, ratio of the chemosensor–Cu complexes was found to be 2 : 1, according to Job plot experiments. 2+ 9 À2 Accepted 24th June 2014 The association constant (Ka) for Cu binding with chemosensor 1 was found to be 9.56 Â 10 M . 2+ DOI: 10.1039/c4nj00695j The maximum fluorescence quenching caused by Cu binding with chemosensor 1 occurred over a pH range of 5–9. Moreover, fluorescence microscopy experiments showed that chemosensor 1 could be 2+ www.rsc.org/njc used as a fluorescent probe for detecting Cu in living cells. 1 Introduction In this study, a new coumarin derivative 1 containing a phenol hydrazone moiety was designed for metal ion detection. The development of highly selective chemosensors for the Two components make up chemosensor 1; a coumarin moiety as a recognition of environmentally and biologically important reporter, and a phenol hydrazone moiety as a metal ion chelator metal ions, such as Cu2+,Cd2+,Fe3+,Hg2+,Pb2+, and Zn2+, (Scheme 1). -
M.Sc. Physical Chemistry Laboratory Manual: CHM 423
M.Sc. Physical Chemistry Laboratory Manual: CHM 423 1st Semester 2012-2013 Venue: Physical Chemistry Laboratory, New Core Building Instructor-in-charge: Dr. Madhav Ranganathan ([email protected]) Lab-in-charge: Budh Prakash Gautam Karmankar Dr. Anjali Pandey Student Tutors: Dipankar Mondal Krishnandu Makhal Indian Institute of Technology Kanpur Kanpur-208016, UP, India CHM 423; 2012-2013 Preface This document covers the necessary basic information you will need in order to complete the laboratory course CHM423. You will be expected to read all of the relevant background material prior to your laboratory. Madhav Ranganathan 28 July 2012 Page: 2 of 56 CHM 423; 2012-2013 Laboratory Introduction Meeting place and times Place: Physical chemistry Laboratory, New Core Building Time: Tuesday and Thursday 2:00 pm - 4:50 pm: LATE ARRIVAL WILL BE PENALIZED. NO ONE WILL BE ALLOWED IN THE LABORATORY AFTER 2:15 pm Instructors : Instructor-in-charge: Dr. Madhav Ranganathan ([email protected]) Office: SL 302 Phone: 6037 Office hours: by appointment Lab-in-charge: Buddha Prakash Gautam Karmankar Dr. Anjali Pandey Student Tutors: Dipankar Mondal Krishnandu Makhal Course goals: To convey the challenges and excitement of Physical Chemistry. In addition to learning various experimental methods, you will be exposed to data collection methods, error analysis and report writing. It reinforces the knowledge you have learned in classes and will give you a chance to apply your knowledge. It will make you familiar how to deal with complexity in experimentation, which is an important component of any scientist’s expertise. Text books There is no text book for this course. -
Interactions Between Spermine-Derivatized Tentacle Porphyrins and the Human Telomeric DNA G-Quadruplex
International Journal of Molecular Sciences Article Interactions Between Spermine-Derivatized Tentacle Porphyrins and The Human Telomeric DNA G-Quadruplex Navin C. Sabharwal 1,2, Jessica Chen 1,3, Joo Hyun (June) Lee 1,4, Chiara M. A. Gangemi 5, Alessandro D’Urso 5,* and Liliya A. Yatsunyk 1,* 1 Department of Chemistry and Biochemistry, Swarthmore College, Swarthmore, PA 19081, USA; [email protected] (N.C.S.); [email protected] (J.C.); [email protected] (J.H.(J.)L.) 2 Lerner College of Medicine, Cleveland Clinic, Cleveland, OH 44195, USA 3 School of Dental Medicine, University of Pennsylvania, Philadelphia, PA 19104, USA 4 College of Dentistry, New York University, New York, NY 10010, USA 5 Department of Chemical Science, University of Catania, 95125 Catania, Italy; [email protected] * Correspondence: [email protected] (A.D.); [email protected] (L.A.Y.); Tel.: +39-095-738-5095 (A.D.); +1-610-328-8558 (L.A.Y.) Received: 16 October 2018; Accepted: 17 November 2018; Published: 21 November 2018 Abstract: G-rich DNA sequences have the potential to fold into non-canonical G-Quadruplex (GQ) structures implicated in aging and human diseases, notably cancers. Because stabilization of GQs at telomeres and oncogene promoters may prevent cancer, there is an interest in developing small molecules that selectively target GQs. Herein, we investigate the interactions of meso-tetrakis-(4-carboxysperminephenyl)porphyrin (TCPPSpm4) and its Zn(II) derivative (ZnTCPPSpm4) with human telomeric DNA (Tel22) via UV-Vis, circular dichroism (CD), and fluorescence spectroscopies, resonance light scattering (RLS), and fluorescence resonance energy transfer (FRET) assays. -
Estimation of Trace Amounts of Chromium (III) in Multi-Vitamin With
ANALYTICAL SCIENCES SEPTEMBER 2004, VOL. 20 1321 2004 © The Japan Society for Analytical Chemistry Estimation of Trace Amounts of Chromium(III) in Multi-Vitamin with Multi-Mineral Formulations Jolly M. SANKALIA, Rajashree C. MASHRU,† Mayur G. SANKALIA, Priti P. PARIKH, and Vijay B. SUTARIYA Pharmacy Department, Faculty of Technology & Engineering, The Maharaja Sayajirao University of Baroda, Kalabhavan, Vadodara, Gujarat 390001, India Two new specific, selective, simple and inexpensive spectroscopic methods for estimating a trace amount of chromium (Cr3+) from a multi-vitamin with multi-mineral pharmaceutical formulations were developed. The proposed methods are based on the conversion of Cr3+ to Cr6+ either by oxidation with a nitric acid–perchloric acid mixture (method I) or by fusion with an excess amount of sodium carbonate (method II), followed by the complexation of Cr6+ with 1,5- diphenylcarbazide (DPC) in a mineral acidic solution of pH 1.0 ± 0.5. The pink-colored complex was estimated at 544 nm. Both methods were found to be linear in the range of 0.1 – 0.8 µg/ml with a limit of detection in the range of 0.0123 – 0.0157 µg/ml and a limit of quantitation in the range of 0.0419 – 0.0525 µg/ml. Method I was found to be suitable for estimating Cr3+ species in various formulations, like tablets, capsules and syrups, while method II was found to be suitable for tablets and capsules. Satisfactory recovery from spiked samples of standard Cr3+ suggests no interference of any excipients and diverse ions present in the formulations. The developed methods were compared with AAS by ANOVA, and no significant difference was observed. -
Interaction Between Calcium and Casein Hydrolysates: Stoichiometry, Binding Constant, Binding Sites and Thermal Stability of Casein Phosphopeptide Complexes
International Dairy Journal 88 (2019) 25e33 Contents lists available at ScienceDirect International Dairy Journal journal homepage: www.elsevier.com/locate/idairyj Interaction between calcium and casein hydrolysates: Stoichiometry, binding constant, binding sites and thermal stability of casein phosphopeptide complexes * Ricardo Troncoso Recio a, , Nelson P. Guerra a, Ana Torrado a, Leif H. Skibsted b a Department of Analytical and Food Chemistry, Faculty of Science, Ourense Campus, University of Vigo, Ourense, Spain b Department of Food Science, University of Copenhagen, Rolighedsvej 30, DK-1958, Frederiksberg C, Denmark article info abstract Article history: The ability of a casein hydrolysate rich in casein phosphopeptides (CPPs) to form calcium complexes was À Received 18 June 2018 studied. An association constant of 125 ± 32 L mol 1 at 25 C was determined electrochemically, forming Received in revised form 1:1 complexes according to conductivity measurements. Chloride anions increased the affinity for cal- 15 August 2018 cium and number of CPPs bound to calcium in solution to more than one. From temperature dependence Accepted 15 August 2018 of the association constant, the enthalpy of binding of calcium chloride to CPPs was determined to Available online 27 August 2018 À be À24 kJ mol 1. Fourier transform infrared spectroscopy confirmed solid state binding of chloride and calcium to CPPs, further showing a positive cooperativity induced by calcium due to opening of the alpha-helix structure of CPPs. In addition, calcium chloride complexation was shown to increase the thermal stability of CPPs according to the results of thermogravimetry differential scanning calorimetry. In conclusion, hydrolysed casein could be considered as a potential enhancer of calcium availability in food.