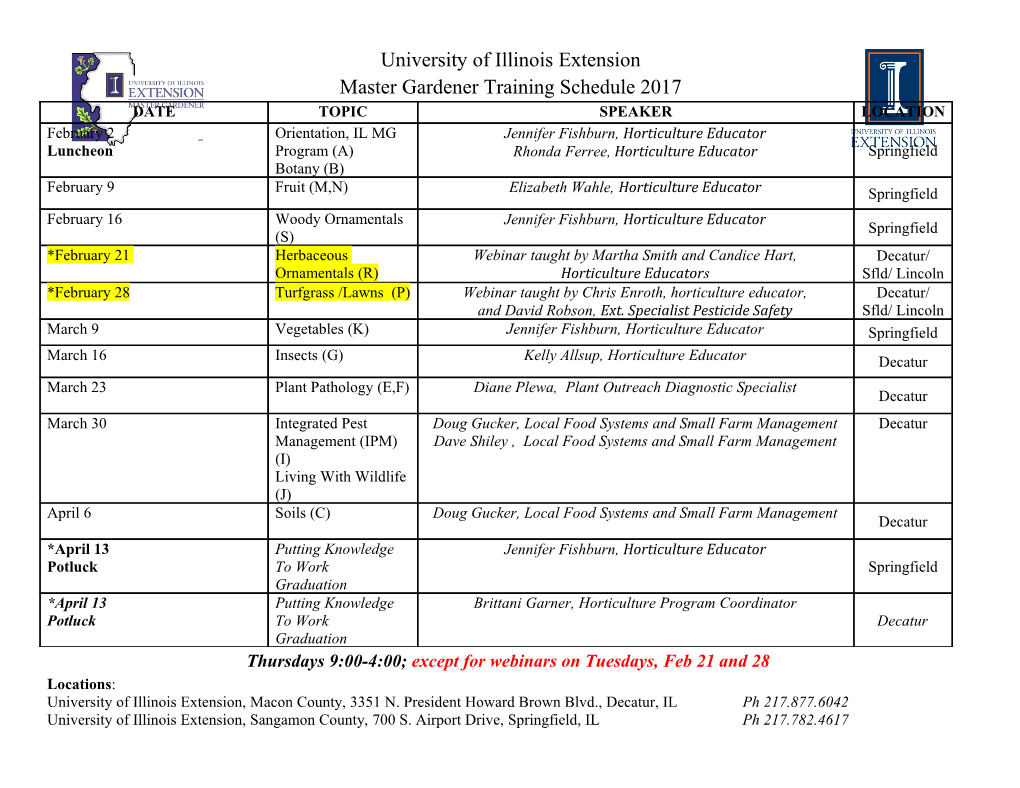
Historical Overview • The field of Elementary Particle Physics has developed in a natural progression since the turn of the last century • This is an attempt to provide the historical context and examples of the experiments that shape our current understanding of the most fundamental principles of nature. • What were some of the major historical developments over the last century? • Interplay between: – Accelerators – Experiments – Theory Radioactivity In 1896 Henri Becquerel accidentally exposed photographic plates to uranium. In 1898 Marie and Pierre Curie isolated polonium and radium (much stronger sources). Henri Becquerel Marie Curie Pierre Curie The Electron In 1897 J.J. Thomson studied “cathode rays” emitted by a hot electrode. Measured deflection by B and E fields. 2 FE = q E, FB = qv x B qvB = m v /rcurv B x r Computed velocity v = E/B using crossed fields, then curv q/m = v/(B rcurv) using B alone. Found very large q/m. Inferred small me. Thomson’s atomic model: • Electron is point-like • At least smaller than 10-17 cm • Like charges repel • Hard to keep electric charge in a small pack ATOMS ARE NOT ELEMENTARY! Quantum Theory • Nils Bohr described atomic • Albert Einstein extends the laws of structure using early concepts classical mechanics to describe of Quantum Mechanics. velocities that approach the speed of light. All matter should obey the laws of quantum mechanics and special relativity. Quantum Theory • Energy and matter are related – Energy can be transformed to mass and vice versa • Conservation of mass-energy • Measured energy of the electron is only 0.5 MeV – Can explain a size of 10-10 to 10-13 cm – Cannot explain < 10-17 cm as measured • Need lots of energy to pack charge tightly inside the electron – Breakdown of theory of electromagnetism • Uncertainty Principle: You can violate energy conservation but only for a short time Werner Heisenberg The Photon Light is a particle: In 1905 Einstein interpreted the photoelectric effect as electron emission due to absorption of a quantum of light: Ee = hν –�. Increasing the light intensity does not increase Ee. Confirmed by Millikan (1916), Compton (1923). Photon energy proportional to frequency: E = h n glass tube under vacuum Current measurement The Nucleus In 1909 Rutherford scattered alpha particles (He nuclei) off a gold foil. One in 8000 scattered at a large angle. Ernest Rutherford “as if you fired a 15-inch naval shell at a piece of tissue paper and the shell came right back and hit you” Evidence that the positive charge in an atom is in a tiny core. Scattering a – atom scattering at low energies is dominated by Coulomb interaction a - particle Atom: spherical distribution impact of electric charges parameter b Geiger & Marsden a – particles with impact parameter = b “see” only electric charge within sphere of radius = b a, b source (Gauss theorem for forces proportional to r-2 ) E. Rutherford Zinc Sulphide Screen 1927, Rutherford, as President of the Royal Society, expressed a wish for a supply of "atoms and electrons which have an individual energy far transcending that of the alpha and beta particles from radioactive bodies..." The Neutron Why did helium have twice the charge of hydrogen, but four times the mass? He = 4 p + 2 e was proposed. In 1928, W. Bothe and H. Becker found that particles from Po cause Be to emit a penetrating radiation, first thought to be photons (γ rays). In 1932 James Chadwick studied ejection of protons from various elements, showed that the neutral particle (n) had a mass very close to mp. Neutron source in Chadwick’s experiments: a 210Po radioactive source (5 MeV a – particles ) mixed with Beryllium powder Þ emission of electrically neutral radiation capable of traversing several centimeters of Pb: 4 9 12 He2 + Be4 ® C6 + neutron ­ James Chadwick a - particle Penetrating Power of Particles a b g Neutron Paper sheet Aluminum Lead Paraffin The Birth of Particle Physics • In 1896, Thomson showed • In 1905, Einstein • In 1907, Rutherford showed that the mass of that electrons were argued that photons particles, not a fluid. an atom was behave like particles. concentrated in a nucleus. Particles that should obey the laws of quantum mechanics and relativity. Nuclear Physics • α, β, ɣ emission • Properties of neutrons • Fission of heavy elements • Nuclear “chemistry” • Nuclear forces • Beta decay • Neutrino postulated • Theories of beta decay Particle Detectors • In 1912, Wilson develops the • Photographic emulsions exposed cloud chamber for seeing the by the passage of charged paths of fundamental particles. particles. Cloud Chamber • Supersaturated Gas • Cloud formation • Used until 1950’s • Condensation started around the ions generated by passing charged particles (ionization), and the resulting droplets were photographed. The Positron electron spin • In 1927, P.A.M. Dirac invented a relativistic wave equation for the electron, containing solutions with electron E = +(p2c2 + m2c4)1/2 and E = -(p2c2 + m2c4)1/2. magnetic dipole moment µe e! -5 • Relativistic Quantum Mechanics µ = » 5.79´10 [eV/T] e 2m – Schrödinger equation e • Not relativistic (space2 but time1) • He interpreted the latter as “filled states”, the “Dirac sea”. • Excitations would have opposite charge but the same 2 mass as electrons. Eg = 2 mec = 1.2 MeV in this case. • Predicted antimatter • You can create more massive objects than you have energy - but they are virtual - i.e., they disappear promptly and rematerialize in particle states that conserve mass-energy • Vacuum is full of quantum bubbles! Paul Adrian Maurice Dirac The Positron • Just as the equation x2=4 can have two possible solutions (x=2 or x=-2), so Dirac's equation could have two solutions, one for an electron with positive energy, and one for an electron with negative energy. 2 2 2 2 2 E – p c = (m0c ) “relativistic invariant” (same value in all reference frames) • Dirac interpreted this to mean that for every particle that exists there is a corresponding antiparticle, exactly matching the particle but with opposite charge. For the electron, for instance, there should be an “anti-electron” called the positron identical in every way but with a positive electric charge. The Positron Carl David Anderson discovered the positive electron (positron) in 1932, for which he won the Nobel Prize for Physics in 1936 Direction of deflection inside a + - magnetic field: it is a positively g rays ® e +e charged particle positron track Equal amounts of matter and antimatter are produced if energy is converted to matter. The Neutrino 1930 Pauli’s neutrino: Energy conservation in beta (b) decay requires the existence of a light, neutral particle n ® p+ + e- + n (e- = b) observed in 1956 210 Radium E: Bi83 (a radioactive isotope produced in the 1 1 0 __0 decay chain of 238U) Wolfgang Pauli n0®p1+e-1+u0 • Large variations in the emission velocities of the b particle seemed to indicate that both energy and momentum were not conserved. • This led to the proposal by Wolfgang Pauli of another particle, the neutrino, being emitted in b decay to carry away the missing mass and momentum. • Neutrinos must be present to account for conservation energy & momentum Theory of β-Decay b- decay: n ® p + e- + n + + 14 14 + b decay: p ® n + e + n (e.g. O8 ® N7 + e + n) ν : the particle proposed by Pauli (named “neutrino” by Fermi) ν : its antiparticle (antineutrino) Enrico Fermi Fermi’s theory: a point interaction among four spin ½ particles, using the mathematical formalism of creation and annihilation operators invented by Jordan. Particles emitted in b – decay need not exist before emission; they are “created” at the instant of decay Prediction of b – decay rates and electron energy spectra as a function of only one parameter: Fermi coupling constant GF (determined from experiments) Discoveries in Cosmic Rays • Era of using cosmic rays for fundamental discoveries begins • Penetrating radiation observed at high altitudes; observation of increase of radioactivity with altitude during a balloon flight • Solutions to Dirac’s equations interpreted as “positive electrons” • Yukawa proposed a “meson” to explain the strong nuclear force • Anderson observed positrons in 1932 and muons in 1936 • In 1912, Viktor Hess investigated • Perkins discovered pions terrestrial radioactivity in balloon experiments. photographic emulsions in 1947 Cosmic Rays Until the late 1940’s, the only existing source of high-energy particles 1932: Discovery of the antiparticle of the electron, the positron (Anderson). Confirmed the existence and prediction that anti-matter does exist (Dirac). 1937: Discovery of the muon (Street and Stevenson). It’s very much like a “heavy electron”. 1947: Discovery of the pion (Powell). Hadron lighter that the proton. Carl Anderson 1936 Nobel Prize Anderson’s experiment: cloud chamber (discovery of the positron) C.D. Anderson, Physical Review 43, 491 (1933) The Nucleus • What is responsible for strong binding between nucleons? • In 1934 H. Yukawa proposed a new light particle, a meson, to carry the strong force between neutrons and protons. • Range of force led to prediction of mmeson = 200 me, “middleweight” between me and mp = 1837 me • New potential which is large at short distances and decreases rapidly at distances larger than about 2 fm. • Treated the problem in a relativistic quantum theory • He clearly showed that in the relativistic quantum world particles interact by exchanging virtual quanta which mediate the force • He predicted the mass of pions Relation between interaction radius & −g2 e−r /a Yukawa Potential, U(r) = s meson mass m: 4π r ! mc2 » 200 MeV R = -13 int mc for Rint » 10 cm Hideki Yukawa Which Meson is it? In 1937 cosmic rays with about the right mass were found. But in 1946 it was shown that the bulk of these particles interact too weakly with nuclei to be Yukawa’s meson.
Details
-
File Typepdf
-
Upload Time-
-
Content LanguagesEnglish
-
Upload UserAnonymous/Not logged-in
-
File Pages105 Page
-
File Size-