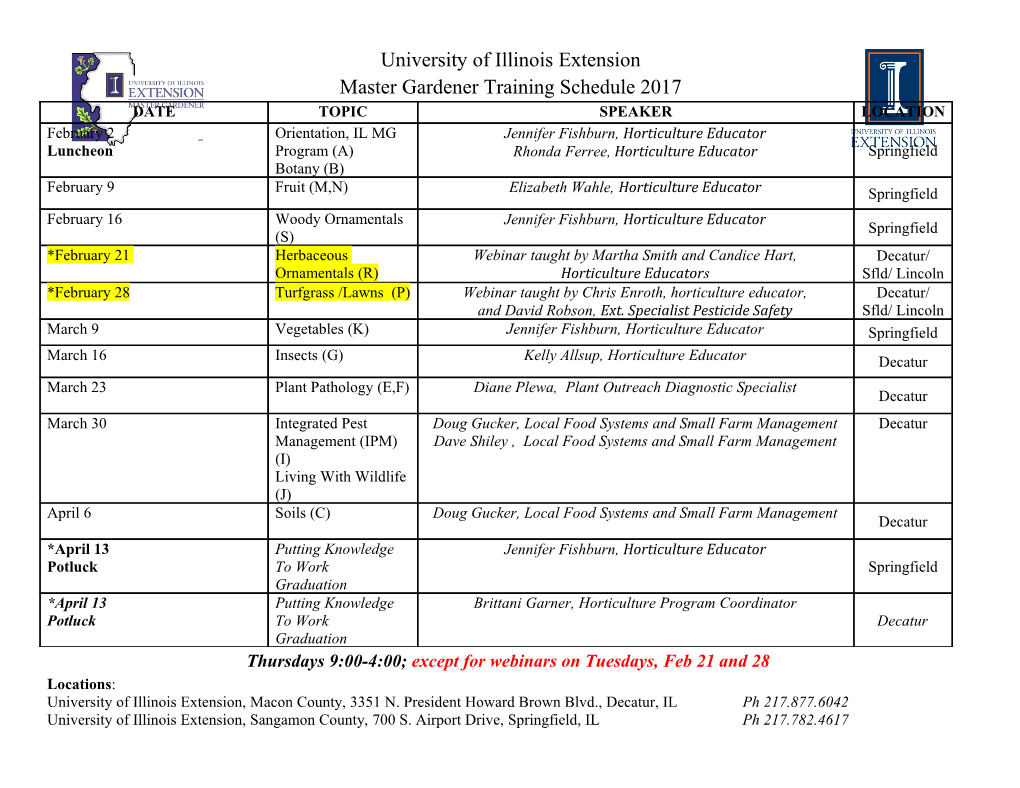
Three-dimensional image reconstruction of dephosphorylated smooth muscle heavy meromyosin reveals asymmetry in the interaction between myosin heads and placement of subfragment 2 Thomas Wendt*, Dianne Taylor*, Kathleen M. Trybus†, and Kenneth Taylor*‡ *Institute of Molecular Biophysics, Florida State University, Tallahassee, FL 32306; and †Department of Molecular Physiology and Biophysics, University of Vermont College of Medicine, Burlington, VT 05405 Communicated by Carolyn Cohen, Brandeis University, Waltham, MA, January 31, 2001 (received for review August 30, 2000) Regulation of the actin-activated ATPase of smooth muscle myosin phorylated form has low ATPase activity, which is greatly II is known to involve an interaction between the two heads that increased on phosphorylation (5). The structural basis of phos- is controlled by phosphorylation of the regulatory light chain. phorylation-dependent regulation in smooth muscle myosin has However, the three-dimensional structure of this inactivated form been studied extensively by using soluble subfragments. Two- has been unknown. We have used a lipid monolayer to obtain headed fragments carrying the full S2 region retain phosphory- two-dimensional crystalline arrays of the unphosphorylated inac- lation-dependent regulation. If the S2 region is shortened, which tive form of smooth muscle heavy meromyosin suitable for struc- weakens dimerization, regulation is lost (6). In addition, single- tural studies by electron cryomicroscopy of unstained, frozen- headed myosin and S1 alone are unregulated (7). These results hydrated specimens. The three-dimensional structure reveals implicate head–head interactions as a fundamental feature of an asymmetric interaction between the two myosin heads. The regulation (8). ATPase activity of one head is sterically ‘‘blocked’’ because part of Although the biochemical effect of smooth muscle myosin II its actin-binding interface is positioned onto the converter domain phosphorylation is well established, the structural basis of reg- of the second head. ATPase activity of the second head, which can ulation is less well understood. Recently, we succeeded in bind actin, appears to be inhibited through stabilization of con- forming two-dimensional arrays of both the phosphorylated and verter domain movements needed to release phosphate and dephosphorylated forms of smooth muscle HMM on a positively achieve strong actin binding. When the subfragment 2 domain of charged lipid monolayer (9). The projection images of arrays of heavy meromyosin is oriented as it would be in an actomyosin dephosphorylated HMM preserved in negative stain revealed an filament lattice, the position of the heads is very different from asymmetric interaction between the two S1 heads. This intramo- that needed to bind actin, suggesting an additional contribution to lecular head–head interaction was abolished when the RLC was ATPase inhibition in situ. thiophosphorylated. This paper reports on the three- dimensional (3D) structure of dephosphorylated smooth muscle phosphorylation ͉ 2-D crystalline arrays ͉ myosin regulation ͉ HMM determined by electron cryomicroscopy of unstained myosin light chains frozen hydrated specimens. The present work facilitated the construction of a considerably better molecular model than was f the 15 types of myosin in the myosin superfamily, only possible from the projection in negative stain, which lacks BIOCHEMISTRY Oisoforms of myosin II are capable of forming filaments (1). information on the relative placement of features perpendicular Myosin II consists of six polypeptide chains, two of which are to the projection direction. The new molecular model reveals the heavy chains that contain actin-binding, ATP catalysis, and proximity of interacting surface loops. The structure also reveals, filament forming activities. Two pairs of light chains, an essential to our knowledge for the first time in a 3D image, a segment of light chain (ELC) and a regulatory light chain (RLC), together the S2 domain in proximity to the two heads, the location of with part of the heavy chain form a lever arm through which which is correlated with earlier electron microscopic images. The force is transmitted to produce filament sliding (2). Myosin II can proposed structure of the inhibited form of smooth muscle be cleaved into several soluble subfragments. Myosin subfrag- myosin is supported by a large volume of mutational experi- ment 1 (S1, the head portion of myosin) contains the ATPase ments, which can now be correlated with a 3D structure. and actin-binding regions of the heavy chain (also called the motor domain) and the light chain lever arm. Subfragment 2 (S2, Materials and Methods the N-terminal portion of the myosin rod), which is predicted to Chicken gizzard smooth muscle HMM was expressed and iso- have an ␣-helical coiled-coil structure (3), links S1 to the lated as described previously (9). Crystallization was carried out filament backbone and forms the myosin heavy chain dimeriza- at 4°C on a positively charged lipid monolayer system (10). tion interface. Another soluble subfragment, heavy meromyosin (HMM), consists of the two S1 heads and S2. Myosin IIs are found in all eukaryotic cells but are most prevalent in muscle Abbreviations: HMM, heavy meromyosin; S1, subfragment 1, the head portion of myosin; S2, subfragment 2, the N-terminal portion of the myosin rod; RLC, regulatory light chain; cells, where they are assembled into an elaborate contractile ELC, essential light chain; 3D, three-dimensional. apparatus. Data deposition: The atomic coordinates have been deposited in the Protein Data Bank, The actin-activated ATPase of vertebrate striated muscle www.rcsb.org (PDB ID code 1I84). myosin II is regulated primarily by proteins bound to the actin ‡To whom reprint requests should be addressed. E-mail: [email protected]. filament. In contrast, the ATPase activity of smooth and non- The publication costs of this article were defrayed in part by page charge payment. This muscle myosin II is regulated by phosphorylation of S19 in the article must therefore be hereby marked “advertisement” in accordance with 18 U.S.C. N-terminal region of the RLC (reviewed in ref. 4). The dephos- §1734 solely to indicate this fact. www.pnas.org͞cgi͞doi͞10.1073͞pnas.071051098 PNAS ͉ April 10, 2001 ͉ vol. 98 ͉ no. 8 ͉ 4361–4366 Downloaded by guest on September 28, 2021 Crystallization buffers consisted of 1 mM Mg2ϩ, 20 mM phos- phate, pH range 7–8, 1 mM ATP, 1 mM EGTA and polyethylene glycol 6000 (7–10%), and NaCl (90–120 mM). Specimens were plunge-frozen for cryoelectron microscopy as described (11) and examined on a Philips (Eindhoven, The Netherlands) CM300- FEG electron microscope at 300 kV accelerating voltage and at ϫ24,000 by using low-dose procedures. Micrographs were screened initially by optical diffraction and digitized on a Perkin–Elmer PDS1010 M microdensitometer at a step size of 0.54 nm with respect to the original object. Micrographs were processed by using the ICE image process- ing software (12). Crystal unbending (13) and merging was done as previously described (9). The 3D reconstruction was obtained by merging the structure factors of 101 images tilted to as high as 60° by standard methods (14). The average unit cell dimen- sions derived from images of untilted specimens are a ϭ 13.3 Ϯ 0.2 nm, b ϭ 30.4 Ϯ 0.9 nm, c ϭ 9.0 nm, and ␥ ϭ 91.5° Ϯ 0.8°, giving a resolution of 2.0 nm. Structure factors were refined to a common phase origin and merged in the two-sided plane group p2. The averaged phase residual was 25°. Model Building. For model building, we constructed an HMM atomic model starting with the x-ray crystal structure of the smooth muscle motor domain plus ELC (15) (PDB ID code 1BR1). Because this structure lacks the RLC, we used the corresponding segments of the x-ray structure of chicken skeletal myosin S1 (PDB ID code 2MYS) as a homology model of this feature (16). For alignment, we used the homologous sequences of the ELC to position the skeletal RLC and missing portions of the heavy chain into the model. We did not construct a homology model for the smooth muscle RLC, which has 55.6% sequence identity with the skeletal RLC. The HMM construct also contains heavy chain residues 853-1175, which comprise the S2 subfragment. This S2 portion was modeled by using an ␣-helical coiled-coil simulation program kindly provided by G. Offer (University of Bristol, Bristol, U.K.) (17). In total, 91 residues for each myosin heavy chain were built into the model to represent the S2 segment. Models were built to fit the elec- tron density by using the x-ray crystallography modeling pro- gram O (18). Results Electron micrographs of the unstained frozen-hydrated two- dimensional crystals of unphosphorylated HMM are virtually featureless, but their Fourier transforms reveal strong diffraction spots to a resolution of Ϸ2.0 nm, which is comparable to the resolution obtained in negative stain (9). Image processing Fig. 1. Two-dimensional arrays of unphosphorylated smooth muscle HMM. showed strong P2 symmetry, indicative of the presence of two (A) Averaged in-plane projection of frozen hydrated arrays with a surface HMM molecules within the unit cell (Fig. 1A). A 2-fold axis view of the 3D reconstruction as Inset (Lower Left). Overlaid on the projection perpendicular to the plane of the crystal relates the pair of HMM is a view of the 3D model reduced to the same scale. The two S1s are colored molecules in the unit cell, but there is no symmetry relationship red and magenta and the S2 cyan. (B and C) Two views of the model fitted to between the two heads within a single molecule. the reconstruction. B is oriented along the crystal a axis. The location of the lipid monolayer, which is not seen in the reconstruction, is drawn to the left The 3D reconstruction reveals a complicated shape, but the of the structure.
Details
-
File Typepdf
-
Upload Time-
-
Content LanguagesEnglish
-
Upload UserAnonymous/Not logged-in
-
File Pages6 Page
-
File Size-