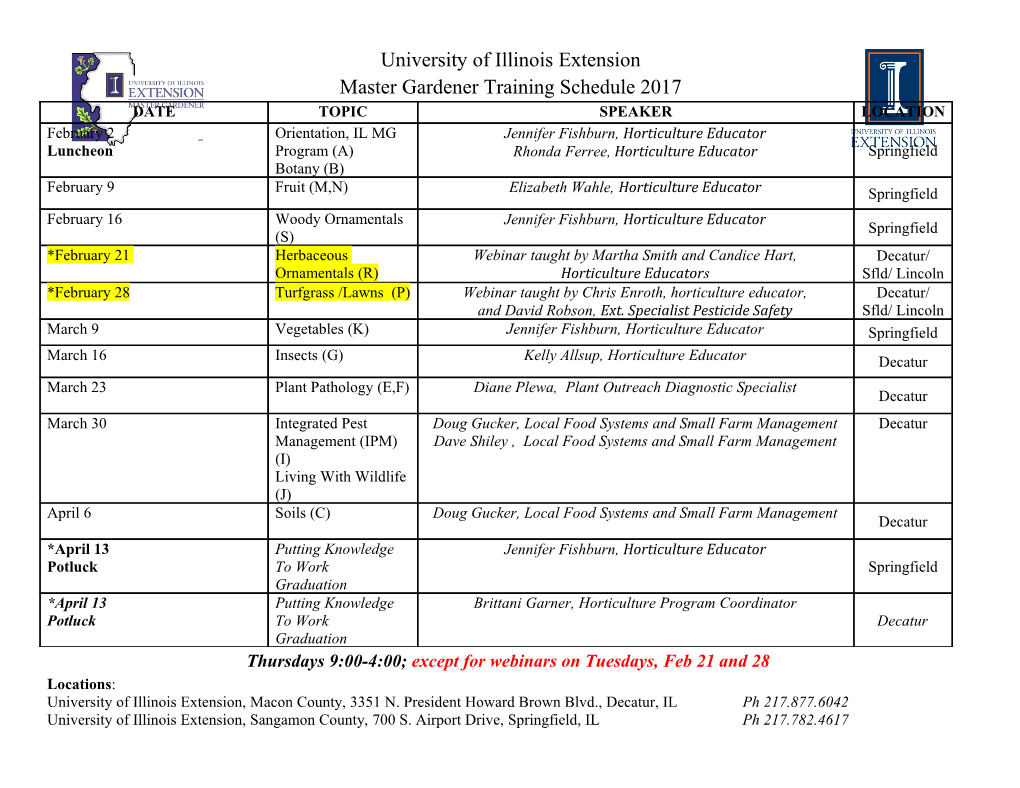
Reviews D. B. Collum et al. DOI: 10.1002/anie.200603038 Lithium Reagents Lithium Diisopropylamide: Solution Kinetics and Implications for Organic Synthesis David B. Collum,* Anne J. McNeil, and Antonio Ramirez Keywords: kinetics · lithium diisopropylamide · metalation · solvent effects · synthesis design Angewandte Chemie 3002 www.angewandte.org 2007 Wiley-VCH Verlag GmbH & Co. KGaA, Weinheim Angew. Chem. Int. Ed. 2007, 46, 3002 – 3017 Angewandte Lithium Diisopropylamide Chemie Lithium diisopropylamide (LDA)is a prominent reagent used in From the Contents organic synthesis. In this Review, rate studies of LDA-mediated reactions are placed in the broader context of organic synthesis in 1. Introduction 3003 three distinct segments. The first section provides a tutorial on solu- 2. Organolithium Solution Kinetics: tion kinetics, emphasizing the characteristic rate behavior caused by A Tutorial 3004 dominant solvation and aggregation effects. The second section summarizes substrate- and solvent-dependent mechanisms that 3. Structure–Reactivity reveal basic principles of solvation and aggregation. The final section Relationships 3007 suggests how an understanding of mechanism might be combined 4. Optimizing Rates and with empirical methods to optimize yields, rates, and selectivities of Selectivities 3013 organolithium reactions and applied to organic synthesis. 5. Summary and Outlook 3015 1. Introduction in all monofunctional solvents.[7–12] Chelating ligands afford isostructural disolvated dimers 2[8b,10] with the notable excep- During a natural product synthesis in 1980, we noted that tion of TMCDA-solvated monomer 3.[8b,13] The structural alkylations of hydrazones displayed odd stereoselectivities control is tactically important because rate studies based on when compared to alkylations of their ketone counter- either uncharacterized reagents or well-characterized mix- parts.[1, 2] Lacking a satisfactory explanation and inspired by tures are of limited value. The structural homogeneity is of Seebachs contemporaneous crystallographic studies of lith- pedagogic value because it allows the nonspecialist to focus ium enolates,[3, 4] we obtained two crystal structures of on reaction coordinates rather than the reactant structures. lithiated hydrazones displaying curious structural features This Review focuses on mechanistic investigations of the that posed more questions than answers.[2] Subsequent rate LDA-mediated reactions summarized in Scheme 1. It is studies led to mechanistic and stereochemical models[5] and, more important, left us captivated by organolithium aggre- gation and solvation. Over the next two decades, we studied a number of synthetically important organolithium reactions with the goal of understanding the mechanistic basis of reactivity and selectivity. Each case study was necessarily prefaced by determining the organolithium structures in solution and was often concluded with computational probes of experimentally elusive details. Solution kinetics, however, provided the compelling insights useful to a broader audience. One reagent has been particularly revealing: lithium diiso- propylamide (LDA). LDA has played a profound role in organic synthesis, serving as the base of choice for a broad range of deproto- nations effected daily by synthetic chemists.[6] LDA is also an ideal template for studying organolithium reactivity. It exists as a single observable structural form—disolvated dimer 1— Scheme 1. Representative reactions mediated by lithium diisopropyla- mide. [*] D. B. Collum, A. J. McNeil, A. Ramirez Department ofChemistry and Chemical Biology Baker Laboratory Cornell University Ithaca, NY 14853-1301 (USA) Fax : (+1)607-255-5223 E-mail: [email protected] Supporting information for this article is available on the WWW under http://www.angewandte.org or from the author. Angew. Chem. Int. Ed. 2007, 46, 3002 – 3017 2007 Wiley-VCH Verlag GmbH & Co. KGaA, Weinheim 3003 Reviews D. B. Collum et al. organized as a series of maxims to underscore the principles Table 1: Relationship ofthe rate law [Eqs. (2) and (3)] to the stoichiom- governing reactivity rather than focus on the mechanistic etry ofthe transition structure. complexity. Section 2offers a tutorial on reaction kinetics, Entry ab kobs Stoichiometry emphasizing the idiosyncrasies caused by solvation and 1/2 À1 ° 1 1/2 À1 k[A2S2] [S] [A(substrate)] aggregation. Section 3 describes general principles of reac- 1/2 0 ° 2 1/2 0 k[A2S2] [S] [AS(substrate)] tivity, many of which we believe are not self-evident. Section 4 1/2 1 ° 3 1/2 1 k[A2S2] [S] [AS2(substrate)] 1/2 2 ° concludes the Review with guidelines that can be dovetailed 4 1/2 2 k[A2S2] [S] [AS3(substrate)] 1/2 3 ° into empirical approaches for optimizing the yields and 5 1/2 3 k[A2S2] [S] [AS4(substrate)] 1 À2 ° selectivities of organolithium reactions. 61À2 k[A2S2] [S] [A2(substrate)] 1 À1 ° 71À1 k[A2S2] [S] [A2S(substrate)] 1 0 ° 810k[A2S2] [S] [A2S2(substrate)] 1 1 ° 911k[A2S2] [S] [A2S3(substrate)] 2. Organolithium Solution Kinetics: A Tutorial 1 2 ° 10 1 2 k[A2S2] [S] [A2S4(substrate)] A picture is worth a thousand words. To a kineticist these pictures come as plots of concentrations versus time and plots below). The pseudo-first-order rate constants (kobs) reveal of observed rate constants versus reagent and solvent how the rates depend on the concentrations of LDA and concentrations.[14] We preface the tutorial on solution kinetics coordinating solvent. Table 2summarizes experimentally with a principle that is as old as kinetics itself. determined rate laws for LDA-mediated reactions to facili- tate access to the primary literature; seven have been 2.1. The Rate Law Provides the Stoichiometry of the Rate- Limiting Transition Structure Relative to the Reactants.[15, 16] Table 2: Experimentally observed mechanisms for LDA-mediated reac- tions (see Scheme 1). The rate law reveals changes in aggregation and solvation Substrate Solvent (S) Stoichiometry[a] Ref. numbers required to reach the rate-limiting transition struc- ° ° ° RBr THF [AS] , [AS2] , [AS3] [33,34a] ture. Therefore, if one has a clear understanding of both the [b] ° ° ° RBr HMPA [AS] , [AS2] , [AS3] [34a] aggregation and the solvation numbers of the reactants, one [c] ° RBr MeOCH2CH2L [AS] [33] [d] ° ° obtains the aggregation and solvation numbers in the rate- RBr PMDTA [AS] ,[A2S] [49] limiting transition structure. RBr TMCDA[d] [AS]° [49] [c] ° ° Rate studies in organolithium chemistry provide reaction RBr MeOCH2CH2L [AS] , [AS2] [43] [b] ° ° enoate HMPA [A2S2] ,[A2S4] [34b] orders and rate laws that are quite diverse. Equations (1)–(3) [c] ° ° epoxide MeOCH2CH2L [AS] ,[A2S] [44] ° ° k epoxide THF [AS] ,[A2S2] [34b] A2S2 þ substrate þ S!product ð1Þ [b] ° epoxide HMPA [A2S2] [34b] ° = t k ester THF [AS2] [25a,b] d½product d ¼ obs½substrateð2Þ [b] ° ° ester HMPA [AS] ,[A2S4] [25a] ° ° a b ester DMPU [AS] , [AS2] [25a] kobs ¼ k½A2S2 ½S ð3Þ ° ester tBuOMe [A2S] [25a] [c] ° ester MeOCH2CH2L [A2S] [48] illustrate a generalized mechanism and rate law. A2S2 is ArH THF [AS]° [29] shorthand for a disolvated dimer in which A refers to the ° ArH nBuOMe [A2S] [29] ° iPr2NLi fragment and S refers to a Lewis basic solvent. imine THF [AS] [9,32b,35a,b] [b] ° Variables a and b refer to their respective reaction orders. imine HMPA [A2S4] [34b] ° ° Table 1 summarizes ten potential mechanisms and affiliated imine TMEDA [AS] ,[A2] [10,35b] ° ° rate laws for LDA-mediated reactions (observable substrate– imine Me2NEt [AS] ,[A2] [35b] LDA complexation, mixed aggregation, and multiple path- [a] Substrate omitted for clarity. [b] THF cosolvent. [c] L =Et, OR, NR2. ways introduce additional variations in rate behavior; see [d] LDA exists as a monomer in TMCDA or PMDTA. David B. Collum received a bachelor’s Anne J. McNeil was born in Buffalo, NY in degree in biology from the Cornell University 1977. She received her BS in chemistry College of Agriculture and Life Sciences in (1999) from the College of William and 1977. After receiving a PhD in 1980 from Mary, where her passion for physical organic Columbia University working with Professor chemistry emerged through her research Clark Still, he returned to the Department with Prof. Robert J. Hinkle. Anne obtained of Chemistry at Cornell, where he is now a her PhD in chemistry from Cornell Univer- Professor of Chemistry. His previous work at sity, where she investigated the structure and Cornell addressed topics in natural products reactivity of lithium enolates derived from b- synthesis and organotransition-metal chemis- amino esters with Prof. David B. Collum. In try, but now focuses on understanding orga- November 2004, she began postdoctoral nolithium structure and mechanism. studies at MIT with Prof. Timothy M. Swager, where she is exploring the properties of encapsulated conjugated polymers. 3004 www.angewandte.org 2007 Wiley-VCH Verlag GmbH & Co. KGaA, Weinheim Angew. Chem. Int. Ed. 2007, 46, 3002 – 3017 Angewandte Lithium Diisopropylamide Chemie documented with frequencies illustrated in Figure 1. We solvent concentration or even a change in solvent dipolarity— routinely refer to these as “idealized rate laws” because tantamount to moving left to right along the x axis—might reaction orders determined by best-fit methods rarely afford cause changes in mechanism and the affiliated rate laws. For integer values. each solvent order there can exist a monomer- and a dimer- based pathway. Thus, for any fixed solvent order there is a competition between monomer- and dimer-based chemistries. 2.2. Rate Constants Are the Currency of Kinetics A typical rate study in our laboratories occurs in two stages. In the first stage, we confront analytical problems and devise protocols for monitoring the reaction. We typically use gas chromatography, in situ IR spectroscopy, or NMR spec- troscopy to monitor reactions.[17] Pseudo-first-order condi- tions are established by setting the substrate as the limiting reagent (Figure 3).[18] Several methods can be used to show Figure 1. Frequency with which the various monomer- and dimer- based mechanisms shown in Table 2 have been observed. Figure 2illustrates a plot of LDA reaction orders versus solvent reaction orders that, although quite odd, is pedagog- ically useful.
Details
-
File Typepdf
-
Upload Time-
-
Content LanguagesEnglish
-
Upload UserAnonymous/Not logged-in
-
File Pages16 Page
-
File Size-