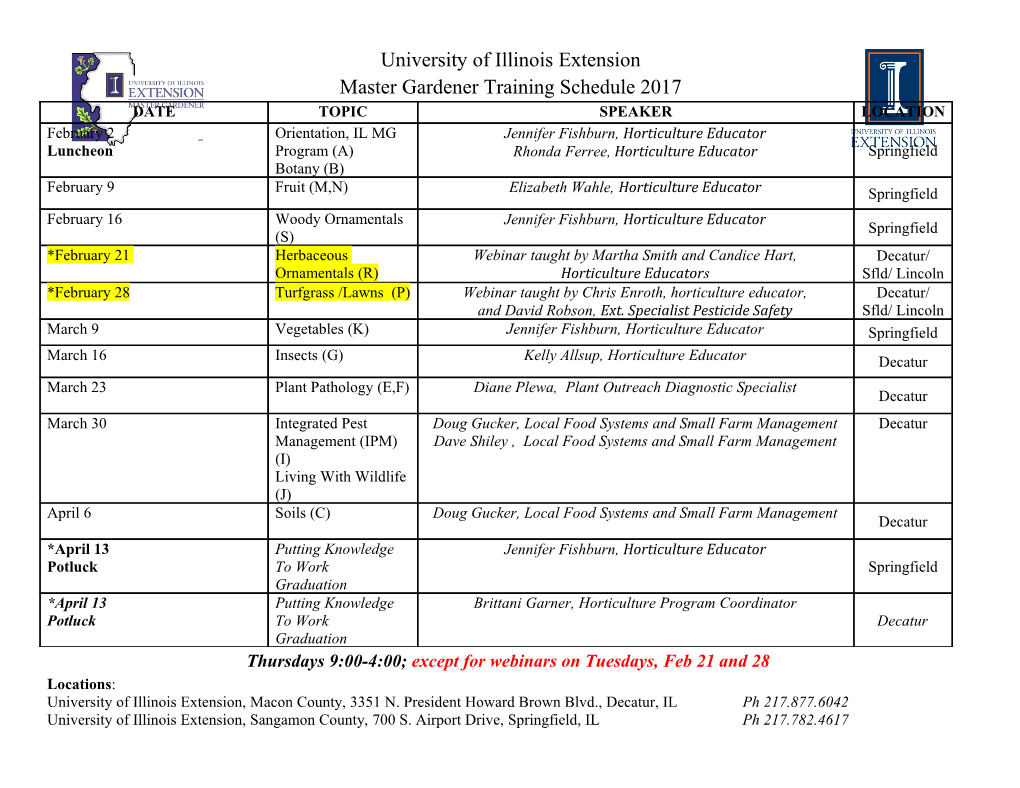
Enzymatic Production of Cellulosic Hydrogen by Cell- free Synthetic Pathway Biotransformation (SyPaB) Xinhao Ye Dissertation submitted to the faculty of the Virginia Polytechnic Institute and State University in partial fulfillment of the requirements for the degree of Doctor of Philosophy In Biological Systems Engineering Y.-H. Percival Zhang, Chair Chenming (Mike) Zhang, Co-Chair Justin Barone Timothy J. Larson July 6, 2011 Blacksburg, Virginia Keywords: biofuel, directed evolution, hydrogen, phosphorylase, protein engineering, rational design, synthetic pathway biotranformation (SyPaB). Copyright 2011 by Xinhao Ye unless otherwise stated Enzymatic Production of Cellulosic Hydrogen by Cell- free Synthetic Pathway Biotransformation (SyPaB) Xinhao Ye ABSTRACT The goals of this research were 1) to produce hydrogen in high yields from cellulosic materials and water by synthetic pathway biotranformation (SyPaB), and 2) to increase the hydrogen production rate to a level comparable to microbe-based methods (~ 5 mmol H2/L/h). Cell-free SyPaB is a new biocatalysis technology that integrates a number of enzymatic reactions from four different metabolic pathways, e.g. glucan phosphorylation, pentose phosphate pathway, gluconeogenesis, and hydrogenase-catalyzed hydrogen production, so as to release 12 mol hydrogen per mol glucose equivalent. To ensure the artificial enzymatic pathway would work for hydrogen production, thermodynamic analysis was firstly conducted, suggesting that the artificial enzymatic pathway would spontaneously release hydrogen from cellulosic materials. A kinetic model was constructed to assess the rate-limited step(s) through metabolic control analysis. Three phosphorylases, i.e. α- glucan phosphorylase, cellobiose phosphorylase, and cellodextrin phosphorylase, were cloned from a thermophile Clostridium thermocellum, and heterologously expressed in Escherichia coli, purified and characterized in detail. Finally, up to 93% of hydrogen was produced from cellulosic materials (11.2 mol H2/mol glucose equivalent). A nearly 20- fold enhancement in hydrogen production rates has been achieved by increasing the rate- limiting hydrogenase concentration, increasing the substrate loading, and elevating the reaction temperature slightly from 30 to 32 oC. The hydrogen production rates were higher than those of photobiological systems and comparable to the rates reported in dark fermentations. Now the hydrogen production is limited by the low stabilities and low activities of various phosphorylases. Therefore, non-biologically based methods have been applied to prolong the stability of α-glucan phosphorylases. The catalytic potential of cellodextrin phosphorylase has been improved to degrade insoluble cellulose by fusion of a carbohydrate-binding module (CBM) family 9 from Thermotoga maritima Xyn10A. The inactivation halftime of C. thermocellum cellobiose phosphorylase has been enhanced by three-fold at 70 oC via a combination of rational design and directed evolution. The phosphorylases with improved properties would work as building blocks for SyPaB and enabled large-scale enzymatic production of cellulosic hydrogen. iii What I cannot create, I do not understand. -- Richard Feynman iv Acknowledgement My time in Virginia Tech has been a gift. I have had the opportunity to work with many great people who have given me much in terms of both personal and professional development. I would like to thank my advisor, Dr. Percival Zhang. Dr. P. Zhang has shaped my life in ways great (enlightening me with his vision) and small (continuously reminding me ‘detail does matter’), some direct (with sharp advices) and some by example (taking fictional characters to be my role models). I’ve tried to rise to the standard he sets, and imagine that I will keep trying for the rest of my scientific lifetime. I am indebted to Dr. Mike Zhang on innumerable levels. He is a generous and calm mentor, a joy to work for, and a friend I can seek suggestions at any time. Without him, I would have given up my Ph.D. study more than a year ago. I am grateful to my committee members, Dr. Justin Barone and Dr. Tim Larson. They always hold the door open for me. Their priceless advice and constructive comments have guided the direction of this research. The research would not be possible without support of the Department of Biological Systems Engineering, Virginia Tech. I really want to express my gratitude to Dr. Saied Mostaghimi and Dr. Mary Leigh Wolfe for their encouragement and support. I also appreciate all the people in BSE for their kind help. I owe a lot to my lab mates. Dr. Jiong Hong and Dr. Wenjin Liu shared many tips and tricks with me and helped solve my problems in molecular biology. I got hand-on experience in enzymology from Dr. Yiran Wang. Despite tense argument, Dr. Xiaozhou Zhang selfishlessly provided his input and suggestions for improving my projects. I’d like to thank Zhiguang Zhu, Noppadon (Tik) Sathitsuksanoh, Joe Rollin, Suwan Myung, Hehuan Liao, and Fangfang Sun for always being there with a warm heart and v sympathetic ears. I am also thankful to Dr. Zuoming Zhang who offered a warm place where I dare to get drunk. My special thanks go to Dr. Jonathan Mielenz, Dao Zhou, Dr. Jean Peccoud, Chuan Han, and Dr. Pengtao Yue. Every phosphorylase studied here was amplified from the genomic DNA of Clostridium thermocellum that is a gift from Dr. Jonathan Mielenz. Dao Zhou designed the printed circuit boards (PCB), through which the hydrogen sensors started to function. The carbohydrate-binding modules (CBM) family 4, family 6, and family 9 in Chapter 4 were synthesized in Dr. Jean Peccoud’s group and given freely. Chuan Han and Dr. Pentao Yue helped me fix the stochastic model in Chapter 5, by which the optimal mutagenesis frequency was identified. I benefit a lot from my former advisors, Prof. Siliang Zhang and Dr. Kean Wang. I’m grateful to their blind investment in me. They opened up my mind and trained me at multiple scales. Much appreciation is given to Gramma (Qilin) Li. I got to know her when I was a sophomore. Since then, she has been the one whom I could contact whenever I had troubles. Another special ‘thanks’ goes to Simon Kwong. He is a mentor for life, a friend in need, and a chef who finds ‘the cure’ to my homesickness. I’d also like to extend my thanks to the crew in Virginia Tech and beyond: Dr. Zheying Guo, Dr. Ruijia Wang, Dr. Zhenyu Zhang, Sarah Jennings and her friends, Dr. Pu Wang, Guanying Wang, Dr. Yihong Yang, Dr. Qinqin Chen, Dr. Souwen Lai, Canming Jiang, Jianzhong Hu, Lifeng Li, Dr. Ying Jin, Dr. Hao Yu, Chuan Han, Dr. Kun Zhou, Cunhao Gao, Senlin Peng, Dr. Zengping Xing, Min Li, Dr. Bin Xu, Dr. Bin Xue, Dr. Shuangchuan Yan, Shuchi Wu, Dr. Liang Shan, Luman Chen, Qin Li, Ian Doran, Yunde Zhang, Dr. Kaigui Bian, Xiaomo Liu, Liguang Xie, Ji Wang, Jian Zhang, Bo Wen, Dr. Yong Wang, and Prof. Damai Zhou, etc. In addition to the science, they have made my life in Blacksburg colorful. Finally, I would like to express my deepest gratitude to my parents for supporting and believing in me as I chase my dreams. I appreciate my sister who takes care of the parents and thus allows me to continue my education abroad. vi Table of Contents Abstract……………………………………………………………………………………ii Acknowledgement……………………………………………….………………….…….v Table of Contents………………………………………………………………………...vii List of Figures…………………………………………………………………………….ix List of Tables……………………………………………………………………………..xi 1. Hydrogen production from carbohydrates: A review…………………………………1 1.1 Introduction…………………………………………………………….………….2 1.2 Carbohydrates-to-hydrogen conversion…………………………………..….……5 1.2.1 Chemical catalysis………………………………………………………...5 1.2.2 Biological catalysis……………………………………………………....10 1.2.3 Newly developed methods………………………………………….……17 1.3 Concluding remarks……………………………………………………….……..21 2. Spontaneous high-yield hydrogen production from cellulosic materials and water catalyzed by enzyme cocktails…………..………………………….………………..22 2.1 Abstract……………………………………………………………………….….23 2.2 Introduction…………………………………………………………….………...23 2.3 Results and Discussion…………………………………………………….…….24 2.4 Experimental Section…………………………………………………………….30 2.5 Supplementary Materials…………………………………………………….…..31 3. Thermophilic α-glucan phosphorylase from Clostridium thermocellum: Cloning, characterization and enhanced thermostability…………………..….…………….…42 3.1 Abstract………………………………………………………………………..…43 3.2 Introduction……………………………………………………………………...43 3.3 Materials and Methods………………………………………………….……….45 3.4 Results and Discussion……………………………………………………….….48 3.5 Conclusion………………………………………………………………….……57 vii 4. Fusion of a family 9 cellulose-binding module improves catalytic potential of Clostridium thermocellum cellodextrin phosphorylase on insoluble cellulose….......58 4.1 Abstract……………………………………………………………….……….....59 4.2 Introduction…………………………………………………………….………...59 4.3 Materials and Methods…………………………………………………………...62 4.4 Results…………………………………………………………………………....65 4.5 Discussion……………………………………………………………………......72 5. Engineering a large protein by combined rational and random approaches: Stabilizing the Clostridium thermocellum cellobiose phosphorylase…………….……….……..76 5.1 Abstract……………………………………………………………….…..……...77 5.2 Introduction…………………………………………………………….….……..77 5.3 Materials and Methods………………………………………………….…….….79 5.4 Results………………………………………………………………….….……..84 5.5 Discussion………………………………………………………….………….....92 5.6 Conclusion………………………………………………………….…………....96 5.7 Supplementary Materials……………………………………………...…………97 6. Conclusion
Details
-
File Typepdf
-
Upload Time-
-
Content LanguagesEnglish
-
Upload UserAnonymous/Not logged-in
-
File Pages141 Page
-
File Size-