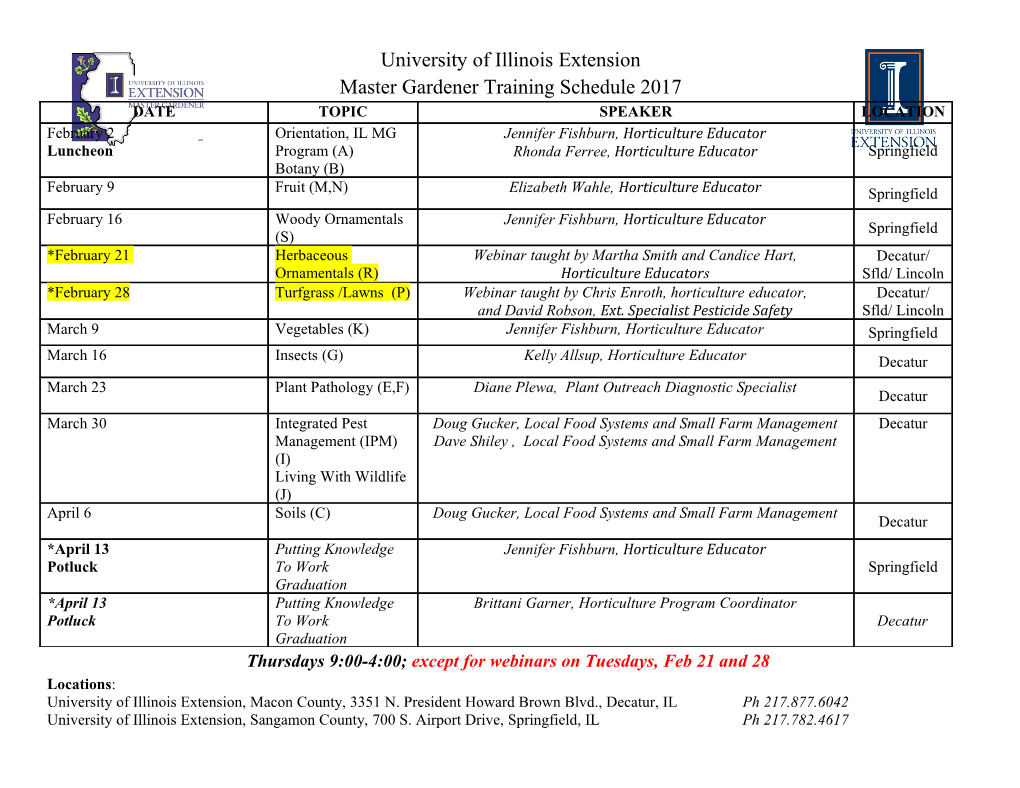
THE ROLE OF THE PARABRACHIAL/KOLLIKER FUSE RESPIRATORY COMPLEX IN THE CONTROL OF RESPIRATION by JOYCE A. BOON B.Sc. Honors The University of Alberta, 1967 M.Sc. The University of British Columbia, 1970 A THESIS SUBMITTED IN PARTIAL FULFILLMENT OF THE REQUIREMENTS FOR THE DEGREE OF DOCTOR OF PHILOSOPHY in THE FACULTY OF GRADUATE STUDIES ZOOLOGY THE UNIVERSITY OF BRITISH COLUMBIA DECEMBER 2004 ©Joyce A. Boon, 2004 Abstract: My goal was to explore the role of the parabrachial/Kolliker Fuse region (PBrKF) of the pons in the production of "state-related" changes in breathing in rats. I hypothesized that the effects of changes in cortical activation state on breathing and respiratory sensitivity are relayed from the pontine reticular formation to the respiratory centres of the medulla via the PBrKF. I found that urethane anaesthetized Sprague Dawley rats spontaneously cycled between a cortically desynchronized state (State I) and a cortically synchronized state (State III), which were very similar to awake and slow wave sleep (SWS) states in unanaesthetized animals, based on EEG criteria. Urethane produced no significant respiratory depression or reduction in sensitivity to hypoxia or hypercapnia. However, breathing frequency (TR), tidal volume (VT) and total ventilation (V TOT) all increased on cortical activation, and changes in the relative sensitivity to hypoxia and hypercapnia with changes in state were similar to those seen in unanaesthetized rats. This indicated that the urethane model of sleep and wakefulness could be used to investigate the effects of cortical activation state on respiration. Since NMDA-type glutamate receptor mediated processes in the PBrKF are known to be important in respiratory control, I examined the role of the PBrKF as a relay site for state effects on respiration by blocking neurons with NMDA-type glutamate receptors with MK-801. I first used systemic blockade and found that it altered resting ventilation and modified the hypoxic, but not the hypercapnic ventilatory response, as has been reported in unanaesthetized animals. Microinjection of MK-801 into the PBrKF confirmed that the 'wakefulness' stimulus for breathing involved glutamate activation of NMDAr on PBrKF neurons, but these neurons were not involved in the response to either continuous or intermittent hypoxia, nor did they change chemosensitivity on cortical activation. However, they indirectly modulated the HVR by altering cortical activation state as animals cycled between State I during hypoxia, and State III post hypoxia. NMDAr in the PBrKF also functioned to return tidal volume to normal following hypoxia and in so doing, prevented the development of long-term facilitation of breathing. iv Table of Contents Abstract: ii Table of Contents iv List of Tables: viii List of Figures ix List of Abbreviations: xiii Acknowledgements: xv Chapter 1 1 General Introduction 1 Introduction 2 Central Organization of Neurons for Respiratory Rhythm and Pattern Generation 3 Respiratory Rhythm Generation 4 Respiratory Pattern Generation 7 Interconnections between Respiratory Related Neurons in the Brainstem 13 The Role of the Respiratory Related Neurons in the PBrKF 14 The Role of Glutamate 17 Location of the NMDA-type Glutamate Receptors 18 Blockade of NMD A Receptors 19 Systemic Injections of Glutamate-receptor Antagonists 19 PBrKF Injections of Glutamate-receptor Antagonists 21 Control of Inspiratory Timing: the Inspiratory Off-Switch (IOS) 21 Effects of Cortical Activation State on Breathing Pattern 22 Anaesthesia, Breathing and the Urethane-Anaesthesia Model of Sleep-Wake 24 Role of the Pons and NMDA-type Glutamate Receptor Mediated Processes in Hypoxic Ventilatory Response 27 Hypotheses 32 References: 34 Chapter 2 46 2.1. Introduction 47 2.2. Methods 49 2.2.1. Animal Care 49 2.2.2. Surgical Preparation 50 2.2.3. Experimental Protocol 50 2.2.4. Data Analysis 52 2.3. Results 53 2.3.1. EEG and Respiratory Traces 53 2.3.2. Effects of cortical activation 56 2.3.3. Effects of hypoxia and hypercapnia 61 2.4. Discussion 66 2.4.1. State Distribution 66 2.4.2. Effects of changes in state on breathing 68 2.4.3. Effects of hypoxia/hypercapnia on breathing 69 2.5. Conclusions 71 2.6. References: 72 Chapter 3 75 3.1. Introduction 76 3.2. Methods 79 3.2.1. Animal Care..... 79 3.2.2. Experimental Protocol 80 3.2.2.1. Surgical Preparation 80 3.2.2.2. Recordings in Animals Breathing Air, 10%O2 in N2 and 5% C02 81 3.2.2.3. Injection of Saline and MK-801 82 3.2.3. Data Analysis -. 82 3.3. Results 84 3.3.1. Respiratory and EEG Traces for Rats Breathing Air 84 3.3.2. Time in State 87 3.3.3. Effects of MK-801 on the EEG 90 3.3.4. Effects of MK-801 on Breathing Pattern 92 3.3.5. Effects of MK-801 on Cortical Activation 96 3.3.6. Effects of MK-801 on the Hypoxic Ventilatory Response 99 3.3.7. The Effect of MK-801 on the Time Domains of the HVR 101 3.3.8. The Effects of MK-801 on the Hypercapnic Ventilatory Response 104 3.3.9. The response of Heart Rate to State Changes and MK-801 106 3.4. Discussion 107 3.4.1. Role of NMDA-type glutamate receptors in determining state 107 3.4.2. Role of NMDA-type glutamate receptors in determining the effects of changes in state on breathing 109 3.4.3. The effects of changes in state on the HVR 110 3.4.4. Role of NMDA-type glutamate receptors in determining the hypoxic ventilatory response Ill 3.4.5. Role of NMDA-type glutamate receptors in determining the hypercapnic ventilatory response and the effects of changes in state on the hypercapnic ventilatory response 113 3.5. Conclusions 114 3.6. References 116 Chapter 4 120 4.1. Introduction: 121 4.2. Methods 124 4.2.1. Animal Care 124 4.2.2. Experimental Protocol: 124 4.2.2.1. Surgical preparation 124 4.2.2.2. Monitoring breathing pattern in air, hypoxia and hypercapnia 125 vi 4.2.2.3. Injection of Saline and MK-801 126 4.2.3. Data Analysis 127 4.2.4. Statistical Analysis: 128 4.3. Results 129 4.3.1. Respiratory and EEG traces 129 4.3.2. Time in State 133 4.3.3. Placement of Injections 135 4.3.4. Effect of MK-801 in rats breathing air 137 4.3.5. Effect of MK-801 on changes in breathing with changes in state 137 4.3.6. Effect of MK-801 in hypoxic rats 141 4.3.7. Effect of MK-801 on the timing of the hypoxic ventilatory response 143 4.3.8. Effect of MK-801 in hypercapnic rats 145 4.4. Discussion: 147 4.4.1. Role of NMDAr in Establishing State 147 4.4.2. Role of NMDAr in the PBrKF in producing changes in breathing with changes in state 148 4.4.3. Role of NMDAr in the PBrKF under resting conditions 149 4.4.4. Role of NMDAr in the PBrKF in the hypoxic ventilatory response and changes in hypoxic sensitivity with changes in state 150 4.4.5. Role of NMDAr in the PBrKF in the hypercapnic ventilatory response and changes in hypercapnic sensitivity with changes in state 152 4.5. Conclusion 153 4.6. References 155 Chapter 5 158 5.1. Introduction: 159 5.2. Methods 162 5.2.1. Animal Care: 162 5.2.2. Experimental Protocol: 162 5.2.2.1. Surgical preparation 162 5.2.2.2. Injection of Saline and MK-801 163 5.2.2.3. Monitoring breathing pattern in air and hypoxia 164 5.2.3. Data Analysis 165 5.2.4. Statistical Analysis: 166 5.3. Results 166 5.3.1. The effect of intermittent hypoxia on cortical activation state and breathing pattern in control rats 166 5.3.2. The effect of intermittent hypoxia on cortical activation state and breathing in MK-801 treated rats 169 5.3.3. The placement of MK-801 injections 169 5.3.4. The time domains of the exposure to intermittent hypoxia with air intervals. ; 173 5.3.5. An examination of the possibility of long-term facilitation of breathing.... 175 5.3.6. The effect of MK-801 injection on the response to intermittent hypoxia.... 177 5.3.7. An analysis of the breathing pattern at critical time points during the intermittent hypoxia exposure 180 vii 5.4. Discussion: 183 5.4.1. The Effects of Intermittent Hypoxia on Cortical Activation State 183 5.4.2. Effects of MK-801 on resting breathing pattern prior to hypoxic exposure. 184 5.4.3. Time domains of the HVR before and after blockade of NMDAr in the PBrKF : 185 5.4.3.1. The Acute Response (AR) 185 5.4.3.2. Short Term Potentiation (STP) 186 5.4.3.3. Short Term Depression (STD) 186 5.4.3.4. Progressive Augmentation (PA) 187 5.4.3.5. Long Term Facilitation (LTF) 187 5.5. Conclusions: 189 5.6 References: 190 Chapter 6 193 6.0 Introduction •. 194 6.1 Preliminary considerations: Urethane anaesthesia 194 6.2 The Role of the PBrKF as a relay for State-related information 198 6.3 The Role of the PBrKF in Chemosensitivity 200 6.4 The Role of the PBrKF in stabilizing the breathing pattern after hypoxia 201 6.5 The Role of the PBrKF in the Generation of Respiratory Rhythm 202 6.6. Summary 203 6.6 References 205 Vlll List of Tables: Chapter 2 Table 2.1 Summary of times of Inspiration and Expiration in States I and III in rats breathing air, 10% 02 or 5% C02 58 Table 2.2 A comparison of the absolute values of fR, VT and V TOT from urethane- anaesthetized rats with those from unanaesthetized rats in a plethysmograph 60 Chapter 3 Table 3.1 Inspiratory and expiratory times before and after MK-801 injection 95 Table 3.2 Heart Rate measured before and after the administration of MK-801 106 Chapter 4 Table 4.1 Mean values ± SEM for Frequency of respiration (fR), Tidal volume (VT), Total ventilation (V TOT), Inspiratory time (TO and Expiratory time (TE) for Sprague Dawley rats breathing air, 10% 02 in N2 or 5% C02 in air 132 Chapter 5 Table 5.1 Mean values ± SEM for fR, VT and V TOT for Sprague Dawley rats before and after injection of MK-801 into the PBrKF 178 IX List of Figures Chapter 1 Fig.
Details
-
File Typepdf
-
Upload Time-
-
Content LanguagesEnglish
-
Upload UserAnonymous/Not logged-in
-
File Pages224 Page
-
File Size-