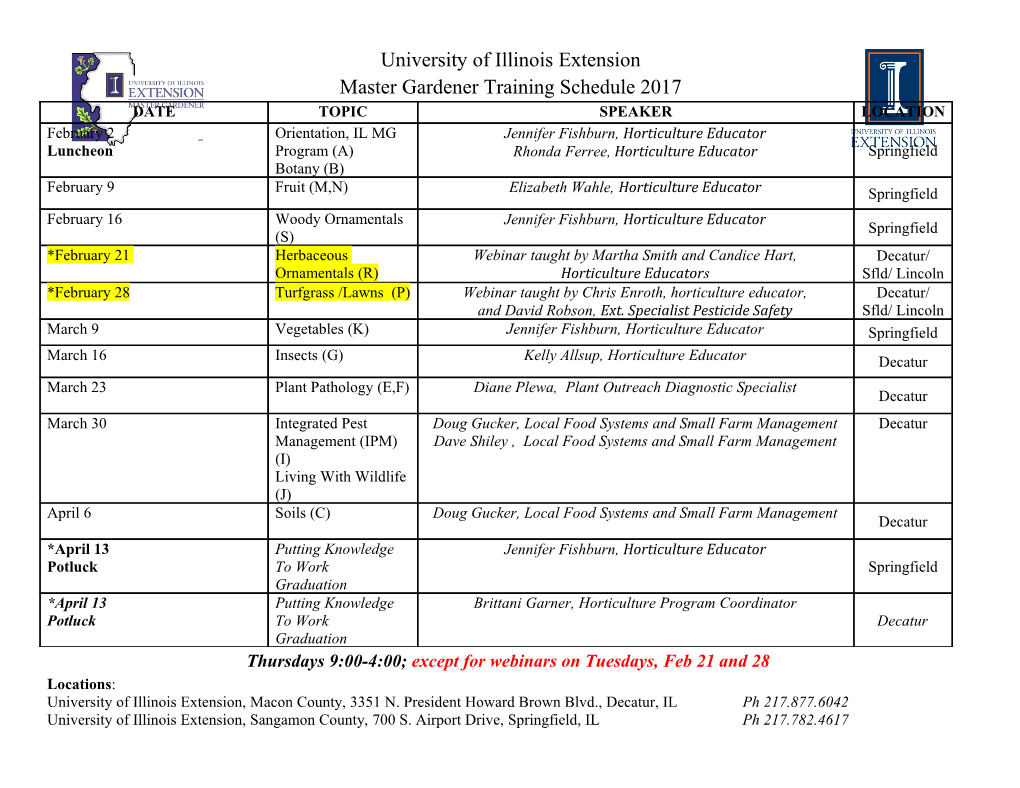
Producing highly complicated materials. Nature does it better Luca Bindi, Massimo Nespolo, Sergey Krivovichev, Gervais Chapuis, Cristian Biagioni To cite this version: Luca Bindi, Massimo Nespolo, Sergey Krivovichev, Gervais Chapuis, Cristian Biagioni. Producing highly complicated materials. Nature does it better. Reports on Progress in Physics, IOP Publishing, 2020, 83 (10), pp.106501. 10.1088/1361-6633/abaa3a. hal-02933187 HAL Id: hal-02933187 https://hal.univ-lorraine.fr/hal-02933187 Submitted on 8 Sep 2020 HAL is a multi-disciplinary open access L’archive ouverte pluridisciplinaire HAL, est archive for the deposit and dissemination of sci- destinée au dépôt et à la diffusion de documents entific research documents, whether they are pub- scientifiques de niveau recherche, publiés ou non, lished or not. The documents may come from émanant des établissements d’enseignement et de teaching and research institutions in France or recherche français ou étrangers, des laboratoires abroad, or from public or private research centers. publics ou privés. 1 Producing highly complicated materials. Nature does it better 2 3 Luca Bindi1,*, Massimo Nespolo2, Sergey V. Krivovichev3,4, Gervais Chapuis5, Cristian 4 Biagioni6 6 1 Dipartimento di Scienze della Terra, Università degli Studi di Firenze, via La Pira 4, I-50121 Firenze, Italy 7 2Université de Lorraine, CNRS, CRM2, Nancy, France 8 3 Department of Crystallography, St. Petersburg State University, University Emb. 7/9, St. Petersburg, 199034, 9 Russian Federation 10 4Nanomaterials Research Center, Kola Science Center, Russian Academy of Sciences, Fersmana str. 14, Apatity, 11 Murmansk region, 184209, Russian Federation 12 5 Institut de Physique, École Polytechnique Fédérale de Lausanne, CH-1015 Lausanne, Switzerland 13 6 Dipartimento di Scienze della Terra, Università di Pisa, Via Santa Maria 53, I-56126 Pisa, Italy 14 15 *Email: [email protected] 16 1 1 2 17 Abstract 18 Through the years, mineralogical studies have produced a tremendous amount of data on 19 the atomic arrangement and mineral properties. Quite often, structural analysis has led to 20 elucidate the role played by minor components, giving interesting insights into the physico- 21 chemical conditions of minerals and allowing the description of unpredictable structures that 22 represented a body of knowledge critical for assessing their technological potentialities. Using 23 such a rich database, containing many basic acquisitions, further steps became appropriate 24 and possible, into the directions of more advanced knowledge frontiers. Some of these 25 frontiers assume the name of modularity, complexity, aperiodicity, and matter organization at 26 not conventional levels, and will be discussed in this review. 27 3 2 4 28 Contents 29 1. Nature: A treasure-trove for structural complexity 30 2. Modularity of crystal structures 31 2.1 Introduction 32 2.2 Symbolic representation of modular structures 33 2.3 Modular structures whose archetype are phyllosilicates 34 2.3.1 Layer modular structures 35 2.3.2 Modular structures with empty interlayer region 36 2.3.3 Modular structures with cations in the interlayer region 37 2.3.4 Modular structures with an O sheet in the interlayer region 38 2.3.5 Rod modular structures 39 2.4 Polyarchetypal modular structures. The example of pyroxenoids 40 3. Information-based analysis of structural complexity of minerals 41 3.1 Introduction 42 3.2 Structural complexity measures 43 3.3 Applications: general overview 44 3.4 Most complex mineral structures 45 3.5 Information-density analysis 46 3.6 Chemical complexity 47 4. Structural complexity, disorder phenomena and the rise of ionic properties 48 4.1 Introduction 49 4.2 NASICON 50 4.3 NaSbO3, brizziite 51 4.4 Ag- and Cu-bearing sulfosalts 52 4.4.1 Pearceite, polybasite and fettelite 53 4.4.2 Argyrodite-canfieldite series 54 5. Structural complexity in sulfosalts and the role of minor constituents 55 5.1 Introduction 56 5.2 Boxwork structures 57 5.3 Other zinkenite-related complex sulfosalts 58 5.4 Information-based complexity in sulfosalts 59 5.5 Role of minor constituents 60 6. Aperiodic minerals 61 6.1 Introduction 62 6.2 Short theoretical presentation 63 6.3 Natrite, Na2CO3 64 6.4 Calaverite Au1-xAgxTe2 2+ 65 6.5 Melilite (Ca,Na)2(Mg,Fe ,Al)[(Al,Si)SiO7] 66 6.6 Natural Quasicrystals 67 6.7 The puzzle of “labradorite” 68 6.8 When “disorder” turns out in aperiodicity 69 7. Conclusions and perspectives 70 Acknowledgements 71 References 72 5 3 6 73 1. Nature: A treasure-trove for structural complexity 74 The study of the materials formed by Nature, i.e. the minerals, has been always the 75 basis for the greatest breakthroughs in chemistry and solid state physics, including the 76 discovery of new elements and chemical compounds, the construction of the periodic table of 77 the elements and the deciphering of the properties of polarized light (Lima-de-Faria 1990). 78 The observations of the mineral morphologies (crystal faces) led to elaborate theories of the 79 structure of solid matter, which were experimentally confirmed in the first decades of the 20th 80 century with the studies on the interaction between X-rays and crystals. The increasingly 81 detailed definition of the physical properties (hardness, tenacity, luminescence, radioactivity, 82 magnetism, electrical and optical properties, etc.) and the compositional and structural 83 characteristics of minerals, as well as the search for natural or synthetic materials with 84 specific mechanical, electrical, magnetic and thermal properties, led to the birth of “materials 85 science” in the middle of the 20th century. The development of materials science was 86 considered as a true technological revolution, a response to precise demands related to 87 economy and industry, and which today is increasingly being used to give answers related to 88 human health and protection of the bio-geosphere. 89 There are about 90 natural chemical elements, which combine to form the ca. 5500 90 known terrestrial and extra-terrestrial mineral species, distinct by chemical composition 91 and/or crystal structure. Minerals represent, in the words of the great Bulgarian mineralogist 92 Ivan Kostov (1913-2004), “...milestones of the history of the Earth and of the Cosmos”, as 93 well as a valuable source of information for research on nanotechnologies and innovative 94 materials. They are the product of natural terrestrial and extra-terrestrial laboratories in which 95 laboratory “tests” have been carried out for ca. 4.8 billion years on thermodynamic systems 96 characterized by wide ranges of chemical-physical parameters and evolutionary processes 97 (temperature, pressure, activity and mobility of chemical components, electromagnetic 98 radiation, compositional, thermal and baric gradients, gravitational field, etc.). 99 Beside the use of natural compounds as technologically-important materials (Ferraris 100 and Merlino 2005; Depmeier 2009), mineral structures with their inherent complexity have 101 also represented a treasure trove for new discoveries in crystallography. For example, in the 102 field of aperiodic crystallography, the mineral calaverite (Au1-xAgxTe2, with 0 ≤ x ≤ 0.33) has 103 been the ideal case to begin to shed light on incommensurability in crystals (Goldschmidt et al 104 1931). Later, mineralogy played an important role with numerous examples of mineral 105 structures exhibiting modularities (Ferraris et al. 2008), fast ion conducting properties (Kuhs 106 and Heger 1979), non-commensurate (misfit) layer structures (Makovicky and Hyde 1981), 107 currently known under the name of composite structures, and in providing the first example of 108 a (billion years old) natural quasicrystal (Bindi et al. 2009c), which settled any doubt that 109 could remain on the long-term stability of quasicrystals. 110 In this review we will focus on different aspects of the complexity of mineral 111 structures, including a quantitative definition of crystal-structure complexity (Krivovichev 112 2013a). 113 114 2. Modularity of crystal structures 115 2.1 Introduction 116 A crystal structure is composed of a very large number of atoms (neutral or ionized) 117 bonded to constitute a three-dimensional, triperiodic network of atoms and of bonds, which 118 can be represented by a graph, where atoms are vertices and bonds are edges. In molecular 119 crystals, the network of bonds can be decomposed into small sub-units (molecules) 120 characterized by stronger bonds (intramolecular bonds), connected by weaker bonds 121 (intermolecular bonds). Moreover, the transition from a state of matter to another (solid to 7 4 8 122 liquid, liquid to gas etc.) preserves the molecular entity: ice, water and water vapour are all 123 characterized by the same molecular component, the H2O molecule, but differ in the way the 124 molecules interact and are arranged one with respect to the other. 125 Inorganic crystals – here we mean really inorganic crystals, not including crystals of 126 metal-organic compounds that, for some inexplicable reason, are today considered in the 127 “inorganic” realm – are mainly non-molecular: they differ from molecular crystals in that 128 bringing them to fusion (or sublimation) does not preserve structural unit common to the 129 states of matter, i.e., a molecule. The largest majority of minerals are non-molecular. Fusion 130 of halite, sphalerite, pyrite, “biotite”, pyrope... (you name it!) does not produce a liquid with 131 intra- and intermolecular bonds. 132 Nevertheless, the structure of inorganic crystals, including minerals, can too be 133 decomposed into elementary building blocks of variable size. Such a decomposition is often – 134 but not always – an interpretation of the crystal structures, rather than a physical reality. The 135 most typical example is the smallest and universally known building block: the coordination 5- 4- 136 polyhedron. An octahedron of NaCl6 or a tetrahedron of ZnS4 do not correspond to 137 chemical units comparable to a molecule: yet, the polyhedral interpretation of inorganic and 138 mineral crystals is the basis of crystal chemistry since its dawn (Pauling 1929; Wells 1975).
Details
-
File Typepdf
-
Upload Time-
-
Content LanguagesEnglish
-
Upload UserAnonymous/Not logged-in
-
File Pages89 Page
-
File Size-