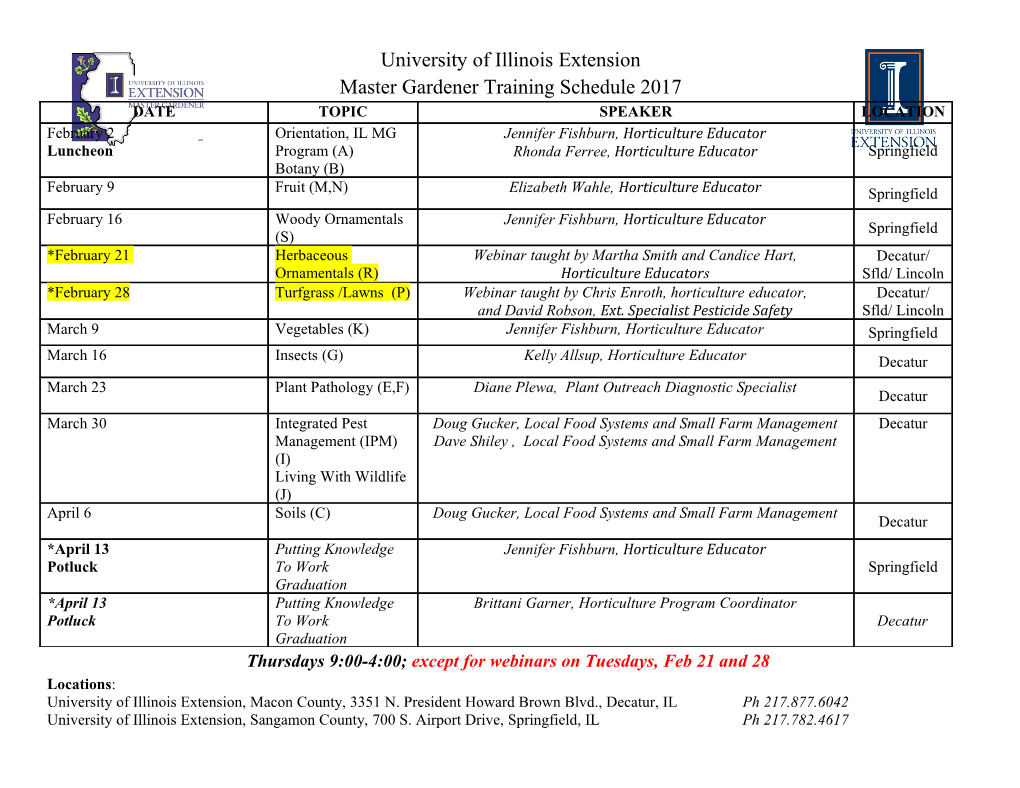
microorganisms Article Insights into Autotrophic Activities and Carbon Flow in Iron-Rich Pelagic Aggregates (Iron Snow) Qianqian Li 1 , Rebecca E. Cooper 1 , Carl-Eric Wegner 1 , Martin Taubert 1, Nico Jehmlich 2 , Martin von Bergen 2,3 and Kirsten Küsel 1,4,* 1 Institute of Biodiversity, Friedrich Schiller University Jena, Dornburger Strasse 159, 07743 Jena, Germany; [email protected] (Q.L.); [email protected] (R.E.C.); [email protected] (C.-E.W.); [email protected] (M.T.) 2 Department of Molecular Systems Biology, Helmholtz Centre for Environmental Research—UFZ, Permoserstrasse 15, 04318 Leipzig, Germany; [email protected] (N.J.); [email protected] (M.v.B.) 3 Pharmacy and Psychology, Faculty of Biosciences, Institute of Biochemistry, University of Leipzig, Brüderstraße 32, 04103 Leipzig, Germany 4 The German Centre for Integrative Biodiversity Research (iDiv) Halle-Jena-Leipzig, Puschstraße 4, 04103 Leipzig, Germany * Correspondence: [email protected]; Tel.: +49-3641-949461 Abstract: Pelagic aggregates function as biological carbon pumps for transporting fixed organic carbon to sediments. In iron-rich (ferruginous) lakes, photoferrotrophic and chemolithoautotrophic bacteria contribute to CO2 fixation by oxidizing reduced iron, leading to the formation of iron-rich pelagic aggregates (iron snow). The significance of iron oxidizers in carbon fixation, their general role in iron snow functioning and the flow of carbon within iron snow is still unclear. Here, we combined a two-year metatranscriptome analysis of iron snow collected from an acidic lake with protein-based Citation: Li, Q.; Cooper, R.E.; 13 stable isotope probing to determine general metabolic activities and to trace CO2 incorporation Wegner, C.-E.; Taubert, M.; Jehmlich, in iron snow over time under oxic and anoxic conditions. mRNA-derived metatranscriptome of N.; von Bergen, M.; Küsel, K. Insights iron snow identified four key players (Leptospirillum, Ferrovum, Acidithrix, Acidiphilium) with relative into Autotrophic Activities and Carbon Flow in Iron-Rich Pelagic abundances (59.6–85.7%) encoding ecologically relevant pathways, including carbon fixation and Aggregates (Iron Snow). polysaccharide biosynthesis. No transcriptional activity for carbon fixation from archaea or eukary- 13 Microorganisms 2021, 9, 1368. otes was detected. CO2 incorporation studies identified active chemolithoautotroph Ferrovum under https://doi.org/10.3390/ both conditions. Only 1.0–5.3% relative 13C abundances were found in heterotrophic Acidiphilium microorganisms9071368 and Acidocella under oxic conditions. These data show that iron oxidizers play an important role in CO2 fixation, but the majority of fixed C will be directly transported to the sediment without feeding Academic Editor: Caroline M. Plugge heterotrophs in the water column in acidic ferruginous lakes. Received: 6 May 2021 Keywords: iron snow; autotrophic iron oxidizing bacteria; heterotrophic iron reducing bacteria; Accepted: 21 June 2021 13 carbon flow; metatranscriptomics; CO2; stable isotope probing; metaproteomics Published: 23 June 2021 Publisher’s Note: MDPI stays neutral with regard to jurisdictional claims in 1. Introduction published maps and institutional affil- iations. Pelagic aggregates, formed in the water column of lakes and oceans through adsorp- tion of inorganic and organic matter (OM), are composed of microorganisms, phytoplank- ton, feces, detritus and biominerals [1,2]. These snow-like aggregates are usually larger than 500 µm and are held together by extracellular polymeric substances (EPS) [3]. Marine pelagic aggregates (marine snow) drive the biological carbon pump via the export of pho- Copyright: © 2021 by the authors. Licensee MDPI, Basel, Switzerland. tosynthetically derived particulate organic carbon (POC) from the photic zone of the ocean This article is an open access article to the deep aphotic zones, where carbon can be sequestered for years before reaching the distributed under the terms and sediment [4–6]. The long residence time of marine snow enables microbial degradation and conditions of the Creative Commons zooplankton grazing activity, which are largely responsible for the attenuation of carbon Attribution (CC BY) license (https:// flux to the deep sea [7]. creativecommons.org/licenses/by/ In iron-rich ferruginous meromictic lakes, iron-rich pelagic aggregates (iron snow) 4.0/). are formed and dominated by an Fe(III)-rich fraction of more than 35%, rather than OM, Microorganisms 2021, 9, 1368. https://doi.org/10.3390/microorganisms9071368 https://www.mdpi.com/journal/microorganisms Microorganisms 2021, 9, 1368 2 of 23 which speeds up their sinking velocity [8]. The world’s largest ferruginous lakes, such as the 600 m deep Lake Matano or Lake La Cruz, serve as analogous representatives to conditions of the Archaean Ocean ecosystems [9]. Here, low productivity in surface water allows sunlight to penetrate to the chemocline, stimulating anoxygenic phototrophic Fe(II)-oxidizing bacteria (FeOB) and anoxygenic green sulfur bacteria to form iron-rich pelagic aggregates [10–13]. In shallow ferruginous lakes, such as the numerous lignite lakes that have emerged in Europe during the last decades [14,15], Fe(II) was or still is provided by groundwater or surface water influx from mine tailings. At low pH conditions, Fe(II) oxidation is mediated mainly by chemolithotrophic acidophilic microbes [16]. The adsorption of phosphorous to the Fe(III) minerals formed within the water column limits primary production by phytoplankton in these lakes [17,18]. These limitations on primary production by phytoplankton suggest that carbon fixation mediated by FeOB might be central for primary production, further trophic interactions within iron snow and carbon export to the sediments. In this study, we sampled iron snow from the model lignite Lake 77 (Lusatian district of Brandenburg, Germany), which also has a meromictic basin [8]. RNA-based quantitative PCR assigned up to 60% of the metabolically active iron snow colonizing microbes to Fe(II)- oxidizing and Fe(III)-reducing bacteria (FeRB) [8,19]. Fe-cycling key players, the FeOB Acidithrix and the FeRB Acidiphilium, isolated from iron snow [20], showed inter-species aggregation controlled by chemical signaling [21]. The heterotrophic Acidiphilium often co-occurs with the chemolithoautotrophic, EPS-producing FeOB Ferrovum, another iron snow key player [22,23]. To capture metabolic activities comprehensively and to follow the flow of carbon 13 within iron snow, we used metatranscriptomics and a CO2 metabolic labeling approach. We collected iron snow below the redoxcline of lignite Lake 77 in two consecutive years (2017 and 2018) to profile the activities of the iron snow microbial community. Mapping mRNA sequences to the genomes of representative Fe-cycling bacteria helped to obtain 13 gene expression profiling. Next, we incubated iron snow with C-CO2 under oxic and anoxic conditions and applied protein-based stable isotope probing (protein-SIP) over time to follow the labeling of peptides in the identified microbial community members. We hy- pothesized that dominant FeOB are mainly responsible for CO2 fixation and polysaccharide biosynthesis and that 13C-labeled organic carbon is rapidly utilized by the heterotrophic FeRB Acidiphilium and Acidocella, as well as other trophic levels. However, our results suggest the majority of fixed CO2 in this system is not feeding other trophic levels in iron snow; instead, the majority of the fixed CO2 is pumped into the sediment. 2. Materials and Methods 2.1. Lake Characteristics and Iron Snow Sampling Lignite Lake 77 is an acidic coal mining lake located in the Lusatian mining area in eastern Germany. Iron snow sampling was conducted in both August 2017 and August ◦ ◦ 2018 at the central basin (CB, 51 108.200 N, 13 41034.700 E) during lake stratification or at the very beginning of the ‘mixing’ period [8]. For lake samples used for Fe(II) and sulfate measurements, 1 mL of 5 M HCl was added to each 5 mL water sample collected from 0–6.5 m depth to prevent abiotic iron oxidation. Iron snow was collected directly onto glass fiber filters (0.9 µm; Infiltec, Speyer, Germany) using an electronic water pump installed between 5 and 6 m depth just below the redoxcline. An volume of 150 L of water was collected in 2017 and 50 L of water were collected in 2018. Glass fiber filters with iron snow were immediately stored on dry ice, transported to the laboratory and stored at −80 ◦C until nucleic acid extraction. For iron snow incubation experiments, additional lake water was collected (20 L of lake water from around 1 m depth and 100 L of water from 5–6 m depth) during the 2018 sampling campaign and stored in 20 L plastic bottles at 4 ◦C until further use. Microorganisms 2021, 9, 1368 3 of 23 2.2. Geochemical Analysis Temperature, pH, conductivity and dissolved oxygen content were measured with a multiparameter meter (YSI, Yellow Springs, OH, USA). Fe(II) concentrations were de- termined spectrophotometrically at 512 nm (Hach Lange, Düsseldorf, Germany) using the phenanthroline method [24]. Sulfate was measured spectrophotometrically at 420 nm, according to the barium chloride–gelatin method [25]. 2.3. Nucleic Acid Extraction of Iron Snow Microbiome A single glass fiber filter with collected iron snow was cut into 12 even pieces, from which two even portions of filter pieces were pooled into 650 mL conical tubes and served as biological replicates. Then, 40–50 mL of oxalate extraction buffer (197
Details
-
File Typepdf
-
Upload Time-
-
Content LanguagesEnglish
-
Upload UserAnonymous/Not logged-in
-
File Pages23 Page
-
File Size-