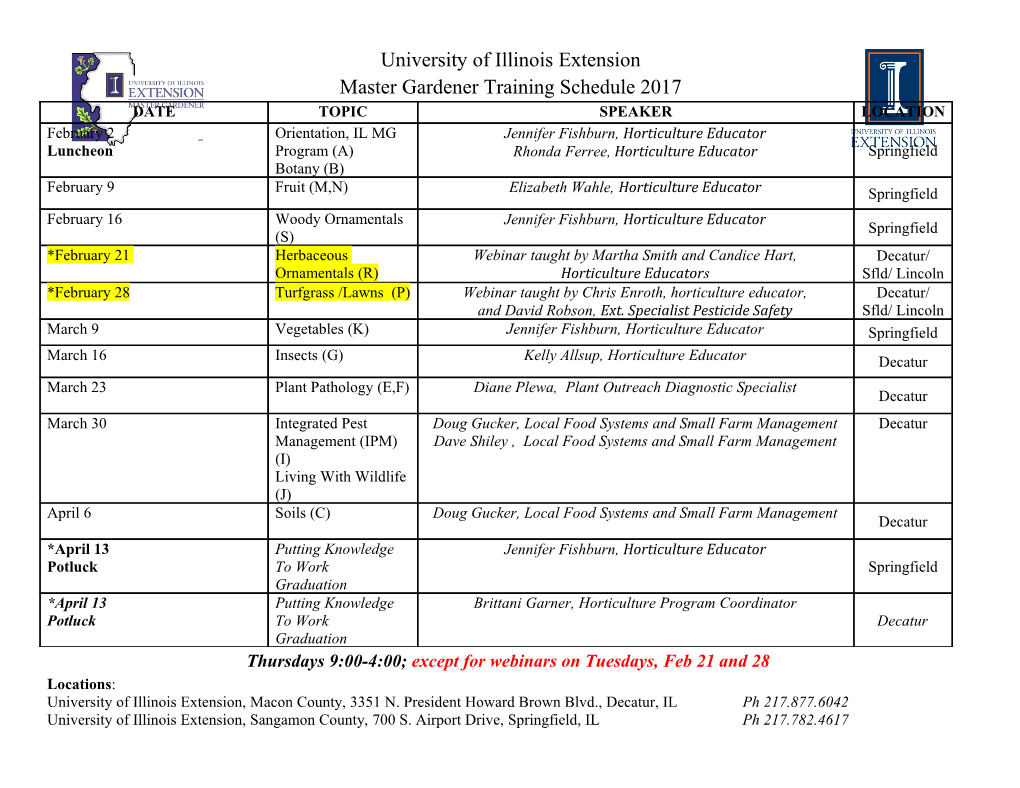
Structure of Trypanosoma brucei flagellum accounts for its bihelical motion Alexey Y. Koyfmana, Michael F. Schmida, Ladan Gheiratmandb, Caroline J. Fua, Htet A. Khanta, Dandan Huangb, Cynthia Y. Heb, and Wah Chiua,1 aNational Center for Macromolecular Imaging, Verna and Marrs McLean Department of Biochemistry and Molecular Biology, Baylor College of Medicine, Houston, TX 77030; and bDepartment of Biological Science and Centre for BioImaging Sciences, National University of Singapore, Singapore 117543 Edited by Wolfgang P. Baumeister, Max-Planck-Institute of Biochemistry, Martinsried, Germany, and approved May 18, 2011 (received for review March 8, 2011) Trypanosoma brucei is a parasitic protozoan that causes African sleeping sickness. It contains a flagellum required for locomotion and viability. In addition to a microtubular axoneme, the flagellum contains a crystalline paraflagellar rod (PFR) and connecting pro- teins. We show here, by cryoelectron tomography, the structure of the flagellum in three bending states. The PFR lattice in straight flagella repeats every 56 nm along the length of the axoneme, matching the spacing of the connecting proteins. During flagellar bending, the PFR crystallographic unit cell lengths remain constant while the interaxial angles vary, similar to a jackscrew. The axo- Fig. 1. Trypanosoma brucei flagellum. (A) Diagram of a trypanosome cell neme drives the expansion and compression of the PFR lattice. (blue) with attached flagellum (yellow). (B) Slice of a tomographic reconstruc- We propose that the PFR modifies the in-plane axoneme motion tion of an isolated straight flagellum. The flagellum, composed of an axo- neme, a PFR (12), and connecting proteins, is attached to the cell body. to produce the characteristic trypanosome bihelical motility as captured by high-speed light microscope videography. PFR is demonstrated by the computed diffraction pattern of a single image (Fig. S2), which shows diffraction spots expected BIOPHYSICS AND rypanosoma brucei has devastated the African continent for for a crystal. In other tomograms, we have observed flagella in COMPUTATIONAL BIOLOGY Tcenturies by infecting humans and domestic animals and bent conformations, similar to those observed during flagellar has hindered economic development in sub-Saharan Africa motion. Because these three flagellar components (PFR, axo- (1). Current sleeping sickness treatments are inadequate and neme, and connecting proteins) exhibit different periodicity and the drugs used are highly toxic (2). In recent years, the motility symmetry, we had to use different strategies to align and average of the T. brucei flagellum has been found to be essential for para- each of them separately (Materials and Methods). The PFR has site survival, infection, and disease pathogenesis (3), and has been found to contain multiple regions (24). We observe these emerged as a promising drug target (4). Flagella with similar distinct regions of the PFR in unaveraged, raw tomogram cross- structural organization and protein composition have also been sections (Fig. S3C and Movie S3). However, in our method of found in euglenoids (5) and other kinetoplastid parasites includ- alignment, the crystallinity of the largest and most well-ordered ing Leishmania spp. and Trypanosoma cruzi, which cause Leish- portion of the PFR (the distal portion) strongly influences the maniasis and Chagas disease, respectively (6). overall PFR average. The proximal PFR region was not observed The trypanosome flagellum is more complex than most other after the averaging because it does not possess such crystallinity. eukaryotic microtubule-based flagella (7–9) and is completely The final averaged structure of the entire flagellum (Fig. 2A different from rotary-motor based bacterial flagella (10). Each and Movie S3) was reconstituted from the averaged components T. brucei cell contains one flagellum that moves the cell body of straight flagella. in an alternating right and left-handed twist resulting in bihelical The averaged PFR density was derived from five different motion (11) (Movie S1). The membrane-enclosed flagellum, tomograms (Fig. 2 A and B). The PFR is a three-dimensional pro- composed of an axoneme, a paraflagellar rod (PFR) (12), and tein lattice that has crisscrossing densities and a large proportion connecting proteins, is attached to the cell body (Fig. 1). PFR of empty volume, both of which are reminiscent of the crystal was identified as a lattice-like ultrastructure in T. brucei flagellum structure of tropomyosin (25, 26). The linear densities are paral- (13). This periodic and crystalline nature of the PFR was con- lel to the crystallographic unit cell axes and may correspond to firmed in T. brucei (14) and related species (15, 16). Monoclonal one or more parallel coiled-coil bundles of the major PFR pro- antibody screens (17) and proteomics studies (18–20) have iden- teins. The diagonal of the crystallographic PFR unit cell repeats tified at least 40 PFR proteins. Among them, PFR1 (73 kDa) and at 56-nm intervals in the direction parallel to the axoneme PFR2 (69 kDa), containing coiled-coil regions (21), are major (Fig. 2B). We used a skeletonization algorithm (27) to provide structural components of the PFR (22). Depletion of these pro- a simplified representation of the densities in the PFR lattice teins results in failure of PFR assembly and cell motility defects (17, 23) (Fig. S1 and Movie S2). In the T. brucei pathogenic blood- Author contributions: A.Y.K., M.F.S., L.G., C.J.F., H.A.K., D.H., C.Y.H., and W.C. designed stream form, ablation of PFR2 causes death of the parasite (18). research; A.Y.K., L.G., C.J.F., H.A.K., and D.H. performed research; A.Y.K., M.F.S., C.Y.H., These results demonstrate a critical role of the PFR in T. brucei and W.C. analyzed data; and A.Y.K., M.F.S., C.Y.H., and W.C. wrote the paper. motility and viability. We have employed cryoelectron tomogra- The authors declare no conflict of interest. phy (cryo-ET) to determine the structure of a biochemically This article is a PNAS Direct Submission. T. brucei isolated flagella (18). We describe here a model that Freely available online through the PNAS open access option. explains how the structure and arrangement of the flagellar Data deposition: The 3D cryoelectron tomographic averages have been deposited in components produces the bihelical motion of the flagellum. the Electron Microscopy Data Bank, www.emdatabank.org (accession nos. EMDB-5302, Fig. 1B is a projection through 30 slices (33 nm) of a tomogram 5303, 5304, 5305, and 5306). of the T. brucei flagellum showing three components of a straight 1To whom correspondence should be addressed. E-mail: [email protected]. flagellum: the crystalline PFR, the axoneme, and the proteins This article contains supporting information online at www.pnas.org/lookup/suppl/ connecting the PFR to the axoneme. The crystallinity of the doi:10.1073/pnas.1103634108/-/DCSupplemental. www.pnas.org/cgi/doi/10.1073/pnas.1103634108 PNAS Early Edition ∣ 1of4 Downloaded by guest on September 25, 2021 similar to axonemes from other organisms (7, 8, 37–40). How- ever, structures such as the outer dynein arms could have been partially removed during the extraction with 1 M KCl. Two rows of connecting proteins (red and pink in Fig. 2 A–C) between the PFR and the axoneme were identified. We observe substantial connections to doublets 5 and 6, but not to 4 and 7 as previously visualized and reported (3, 41). The connections to doublets 4 and 7 are not as bulky as those to doublets 5 and 6. They are longer and perhaps more flexible, and therefore not readily visible in the raw tomograms. Indeed it was shown that connections to doublets 4 and 7 are thin linear structures (13, 14, 16, 24, 42). Due to the difficulty in visualizing the con- nectors to doublets 4 and 7, they were not chosen for averaging from our reconstructions. The connecting proteins which we do observe (to doublets 5 and 6) repeat every 56 nm in straight flagella, a distance corresponding to seven tubulin dimers along the axoneme (Fig. 2B). This distance is the same as the PFR repeat along the axoneme and also similar to periodic attach- ments seen in the Euglena axoneme (5). Each of the two rows of connecting proteins was averaged along the length of the flagella. The averages of the connecting proteins in the two rows are similar in size (Fig. 2B and Movie S3) and are schematically represented by spheres in Fig. 2C. It is likely that each connecting density is a complex of several proteins. Fig. 2. T. brucei flagellum components. (A) Flagellum cross-section. The ax- Whereas straight flagella were used to determine the average oneme is radially colored: central pair complex (yellow), radial spokes (light structure, bent flagella can suggest a model for their motion. Fig. 3 green), microtubule doublets (blue). The dashed red line (bisecting the cen- A–F tral pair) represents the plane of bending of an axoneme. The PFR is offset show top and side views of tomogram slices of the PFR from from that plane (dashed purple line through the middle of the PFR). (B) The three differently bent flagella. The PFR averages are shown in diagonal (shown by a horizontal white arrow) of the crystallographic unit cell different colors. The crystallographic unit cell lengths of bent of the PFR (green) repeats with the same spacing (56 nm) as that of the and straight PFR agree within 5%: a ¼ 52 Æ 2, b ¼ 46 Æ 2, and connecting proteins (red and pink) along the axoneme axis. (C) The layered c ¼ 22 Æ 1 nm (a total of 7,000 unit cells went into these nature of the PFR. Skeletonized (27) PFR density is shown in gray, the crystal- averages) (Fig. 3G, Fig.
Details
-
File Typepdf
-
Upload Time-
-
Content LanguagesEnglish
-
Upload UserAnonymous/Not logged-in
-
File Pages4 Page
-
File Size-