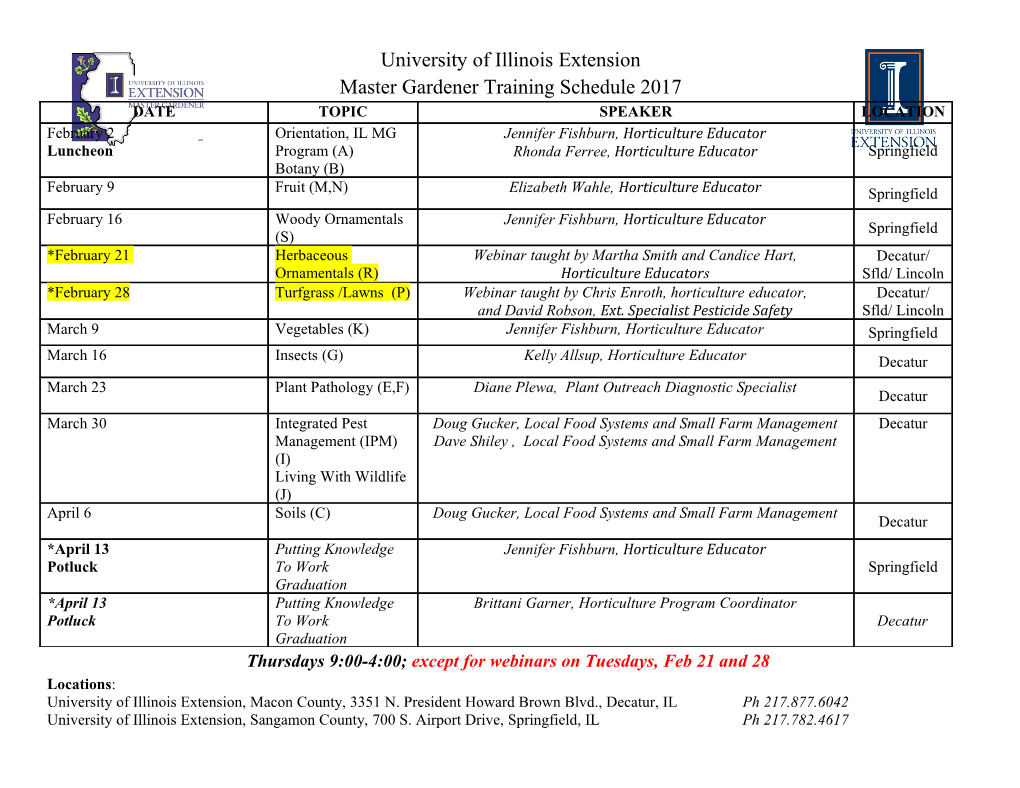
View metadata, citation and similar papers at core.ac.uk brought to you by CORE ORIGINAL RESEARCH ARTICLE published:provided 18 June by 2012 Frontiers - Publisher Connector doi: 10.3389/fmicb.2012.00221 Modeling the habitat range of phototrophs inYellowstone National Park: toward the development of a comprehensive fitness landscape Eric S. Boyd 1*, Kristopher M. Fecteau 2, Jeff R. Havig 3, Everett L. Shock 2,3 and John W. Peters 1 1 Department of Chemistry and Biochemistry, Astrobiology Biogeocatalysis Research Center, Montana State University, Bozeman, MT, USA 2 Department of Chemistry and Biochemistry, Arizona State University, Tempe, AZ, USA 3 School of Earth and Space Exploration, Arizona State University, Tempe, AZ, USA Edited by: The extent to which geochemical variation shapes the distribution of phototrophic metab- Martha Gledhill, University of olisms was modeled based on 439 observations in geothermal springs in Yellowstone Southampton, UK National Park (YNP), Wyoming. Generalized additive models (GAMs) were developed to Reviewed by: Alan Angelo DiSpirito, Iowa State predict the distribution of phototrophic metabolism as a function of spring temperature, University, USA pH, and total sulfide. GAMs comprised of temperature explained 38.8% of the variation James F.Holden, University of in the distribution of phototrophic metabolism, whereas GAMs comprised of sulfide and Massachusetts Amherst, USA pH explained 19.6 and 11.2% of the variation, respectively. These results suggest that of *Correspondence: the measured variables, temperature is the primary constraint on the distribution of pho- Eric S. Boyd, Department of Chemistry and Biochemistry, totrophs in YNP. GAMs comprised of multiple variables explained a larger percentage of Astrobiology Biogeocatalysis the variation in the distribution of phototrophic metabolism, indicating additive interactions Research Center, Montana State among variables. A GAM that combined temperature and sulfide explained the greatest University, 103 Chemistry Research variation in the dataset (53.4%) while minimizing the introduction of degrees of freedom. In Building, Bozeman, MT 59717, USA. e-mail: [email protected] an effort to verify the extent to which phototroph distribution reflects constraints on activity, we examined the influence of sulfide and temperature on dissolved inorganic carbon (DIC) uptake rates under both light and dark conditions. Light-driven DIC uptake decreased sys- tematically with increasing concentrations of sulfide in acidic, algal-dominated systems, but was unaffected in alkaline, cyanobacterial-dominated systems. In both alkaline and acidic systems, light-driven DIC uptake was suppressed in cultures incubated at temper- atures 10˚C greater than their in situ temperature. Collectively, these quantitative results indicate that apart from light availability, the habitat range of phototrophs in YNP springs is defined largely by constraints imposed firstly by temperature and secondly by sulfide on the activity of these populations that inhabit the edges of the habitat range.These findings are consistent with the predictions from GAMs and provide a quantitative framework from which to translate distributional patterns into fitness landscapes for use in interpreting the environmental constraints that have shaped the evolution of this process through Earth history. Keywords: photosynthesis, sulfide, temperature, fitness landscape, CO2 uptake and fixation, habitat range, landscape ecology, distribution INTRODUCTION historical constraints on the evolution of a particular metabolic The distribution of organisms and the functions that they cat- function as imposed by the environment (Wiens and Graham, alyze on Earth today is rooted, at least in part, to the numerous 2005; Westoby, 2006; Boyd et al., 2010; Hamilton et al., 2011a,b). adaptations that enable life to radiate into new ecological niches Such observations can in turn be used to predict the response that have played out over evolutionary time. Such responses are of populations or metabolic guilds to changing environmental recorded in extant organismal distribution patterns (e.g., habi- conditions (Keddy, 1992; Lavorel and Garnier, 2002; Guisan and tat range), as well as in the genetic record of organisms. This Thuiller, 2005). is a consequence of the predisposition for microorganisms to The extreme variation in the geochemical composition of acquire their ecological traits through vertical inheritance, a phe- present day environments is likely to encompass those that were nomenon that manifests in a positive relationship between the present on early Earth (Shock and Holland, 2007), when key meta- ecological relatedness of organisms and their evolutionary related- bolic processes such as photosynthesis are thought to have evolved. ness (niche conservatism; Wiens, 2004; Wiens and Graham, 2005). Yellowstone National Park (YNP), Wyoming harbors >12,000 Thus, extant patterns in the distribution of species or metabolic geothermal features that vary widely in temperature and geochem- function offer “a window into the past” and can be used to infer ical composition, both spatially and temporally (Nordstrom et al., www.frontiersin.org June 2012 | Volume 3 | Article 221 | 1 Boyd et al. Modeling the habitat range of photosynthesis 2005; Shock et al.,2010). Such environments provide a field labora- MATERIALS AND METHODS tory for examining the tendency for guilds of organisms to inhabit PREDICTIVE MODELING particular ecological niches and to define the range of geochemical The binary distribution (presence/absence) of genes involved in conditions tolerated by that functional guild (i.e., habitat range or chlorophyll biosynthesis and/or phototrophic pigments in a num- zone of habitability; Hoehler, 2007; Shock and Holland, 2007). We ber of geothermal springs sampled from across YNP (Figure 1) assume that since it is unlikely that a metabolic process emerged and the associated total sulfide, pH, and temperature of the spring under environmental conditions that no longer support that func- water was extracted from three previous studies (Boyd et al., 2010; tion, such information can help quantify the habitat range for a Cox et al., 2011; Hamilton et al., 2011b). The dataset extracted metabolic process, and can provide insight into the characteristics from Cox et al.(2011) was based on the visual distribution of of an environment that enabled the adaptation of that process into pigments in geothermal springs, whereas the datasets extracted new habitats. from Boyd et al.(2010) and Hamilton et al.(2011b) was based on Phototrophy is the utilization of solar energy by plants, algae, the presence/absence of the protochlorophyllide reductase sub- and certain bacteria to generate energy for the synthesis of complex unit L gene (chlL/bchL) required to synthesize chlorophyll in both organic molecules (Blankenship, 1992; Chew and Bryant, 2007). A anoxygenic and oxygenic phototrophs (Chew and Bryant, 2007) number of recent studies have documented non-random pattern in biomass sampled from geothermal springs in YNP. While the in the distribution of phototrophic assemblages along geochem- data extracted from each of the aforementioned studies were from ical gradients in YNP, Wyoming (Boyd et al., 2010; Cox et al., observations made in different springs from different regions dur- 2011; Hamilton et al., 2011b). These studies qualitatively iden- ing different years, plots of the overall phototroph distribution tified three ecological axes (temperature, pH, and total sulfide) trends with respect to pH, temperature, and sulfide as presented in that appear to constrain the habitat range of phototrophs in all three manuscripts (Boyd et al., 2010; Cox et al., 2011; Hamilton the geothermal features of YNP. However, the extent to which et al., 2011b) and in Figures 2A,B revealed the same trends, with each environmental parameter, or combinations therein, shape an upper temperature limit for photosynthesis of s73˚C and a the distribution of phototrophic metabolism in YNP is unclear. pH-dependent suppression of the upper temperature limit below Moreover, it is unclear if the qualitative trends in the distribu- pH s6. Moreover, the distribution of phototrophic metabolism tion of phototrophic metabolism in YNP noted previously are was constrained to environments with sulfide at a concentration the result of the negative effects that temperature, pH, and sul- of <5 mM(Cox et al.,2011; Hamilton et al.,2011b). Together,these fide have on the activity of phototrophic populations, which observations indicate that both methods, as described above, were in turn would be expected to decrease fitness and limit their suitable for mapping the habitat range of phototrophic metabo- distribution. lism along ecological gradients in YNP.By combining the datasets, Here, in an effort to better define the basis for the observed we were able to sample a much greater portion of the YNP geot- habitat range of phototrophs in YNP,we compiled environmental hermal complex that comprised more than 439 observations, than metadata and presence/absence distributional data from several- any one dataset provided alone. independent examinations of phototrophic metabolisms across Binomial GAMs describing the distribution of phototrophic the YNP geothermal complex (Boyd et al., 2010; Cox et al., pigments and genes involved in their synthesis as proxies for pho- 2011; Hamilton et al., 2011b). This binary dataset comprising totrophs were generated using the mgcv package (Wood, 2011) 439-independent observations
Details
-
File Typepdf
-
Upload Time-
-
Content LanguagesEnglish
-
Upload UserAnonymous/Not logged-in
-
File Pages11 Page
-
File Size-