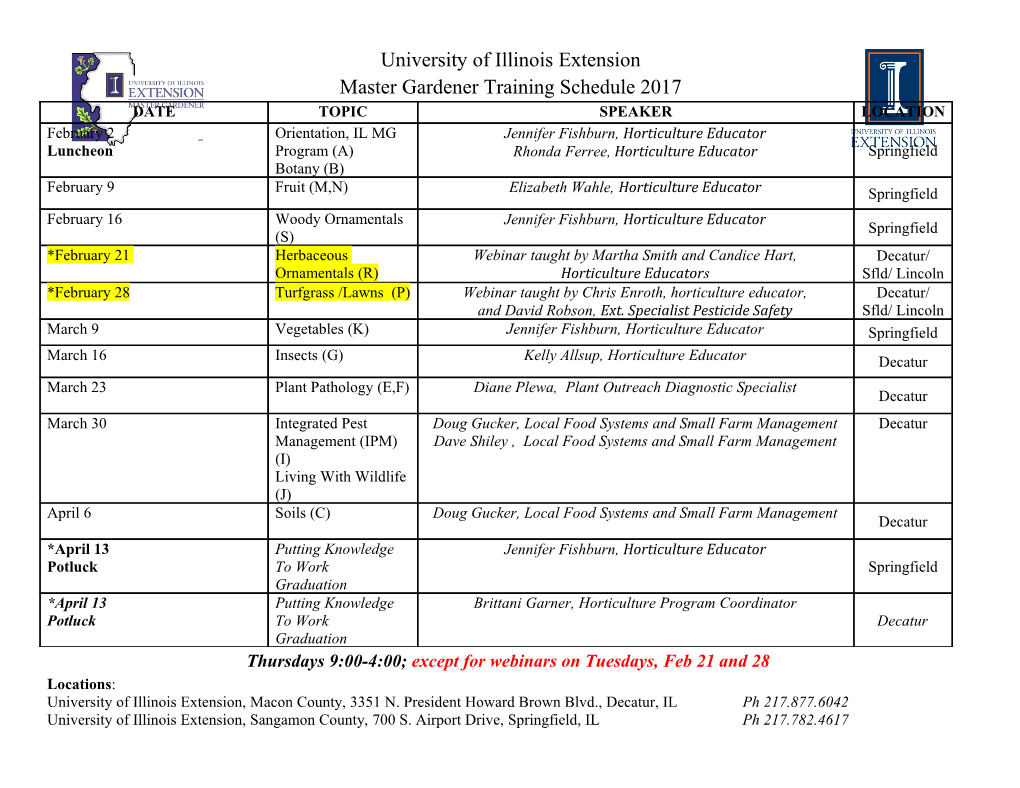
Manoeuvring model of an estuary container vessel with two interacting Z- drives Guillaume Delefortrie, Manasés Tello Ruiz & Marc Vantorre Journal of Marine Science and Technology Official Journal of the Japan Society of Naval Architects and Ocean Engineers (JASNAOE) ISSN 0948-4280 J Mar Sci Technol DOI 10.1007/s00773-017-0508-0 1 23 Your article is protected by copyright and all rights are held exclusively by JASNAOE. This e-offprint is for personal use only and shall not be self-archived in electronic repositories. If you wish to self-archive your article, please use the accepted manuscript version for posting on your own website. You may further deposit the accepted manuscript version in any repository, provided it is only made publicly available 12 months after official publication or later and provided acknowledgement is given to the original source of publication and a link is inserted to the published article on Springer's website. The link must be accompanied by the following text: "The final publication is available at link.springer.com”. 1 23 Author's personal copy Journal of Marine Science and Technology https://doi.org/10.1007/s00773-017-0508-0 ORIGINAL ARTICLE Manoeuvring model of an estuary container vessel with two interacting Z-drives Guillaume Delefortrie1 · Manasés Tello Ruiz2 · Marc Vantorre2 Received: 26 July 2017 / Accepted: 18 November 2017 © JASNAOE 2017 Abstract A 6 degrees of freedom manoeuvring model of an estuary container vessel was implemented in the inland navigation simula- tor Lara at Flanders Hydraulics Research (FHR). The container vessel, with dimensions 110 m × 17.5 m × 4.5 m, is equipped with two Z-drives, each one consisting of two contra rotating propellers. Both Z-drives have a 360° azimuth angle of opera- tion during manoeuvring. The mathematical manoeuvring model was built to cover all degrees of operation. This required the execution of a significant number of captive model tests in the shallow water towing tank of FHR (in co-operation with Ghent University). Based on these tests a new mathematical manoeuvring model was built to cover all effects of operation, including the interaction effects between the two Z-drives. The new mathematical model was implemented in the simulator and both fast time and real time manoeuvring simulations have been carried out. A paper discussing the validation of the manoeuvring model has been presented during MARSIM 2015 (Vos, Delefortrie, and Van Hoydonck in Validation of the manoeuvring behaviour on an estuary vessel. MARSIM, Newcastle, 2015). The present paper discusses the details of the manoeuvring model, with emphasis on the interaction effects between the Z-drives and the influence on the hydrodynamic forces. Keywords Manoeuvring · Shallow water · Thruster interaction List of symbols GM(T) Transverse metacentric height above centre of a Flow acceleration factor (−) gravity (m) aH Additional steering induction for Y (−) GML Longitudinal metacentric height above centre of 2 AR Rudder area (m ) gravity (m) AEP Expanded area ratio of propeller (−) I Moment of inertia (kg m2) 2 AW Water plane area (m ) J Propeller advance (−) B Ship breadth (m) K(*) Roll moment [derivative of * (* represents any CB Block coefficient (−) combination of kinematical parameter(s) u, v, w, CD Drag coefficient (−) p, q, r or their derivatives)] (Nm) CL Lift coefficient (−) KT Thrust coefficient (−) CT Thrust coefficient (−) KM Transverse metacentric height above keel (m) D Ship depth; propeller diameter (m) L Lift in drive bound axis system (N) D Drag in drive bound axis system (N) LD Duct length (m) DDEN Duct entry diameter (m) LOA Ship length over all (m) DDEX Duct exit diameter (m) L(PP) Ship length between perpendiculars (m) F Force (N) m Ship’s mass (kg) M(*) Pitch moment [derivative of * (* represents any combination of kinematical parameter(s) u, v, w, * Guillaume Delefortrie p, q, r or their derivatives)] (Nm) [email protected] N Propeller rate (rpm, 1/s) 1 Flanders Hydraulics Research, Berchemlei 115, N(*) Yawing moment [derivative of * (* represents any 2140 Antwerp, Belgium combination of kinematical parameter(s) u, v, w, 2 Maritime Technology Division, Ghent University, p, q, r or their derivatives)] (Nm) Technologiepark Zwijnaarde 904, 9052 Ghent, Belgium O Origin of coordinate system (−) Vol.:(0123456789)1 3 Author's personal copy Journal of Marine Science and Technology p Roll velocity (rad/s) i Interaction; summation P Propeller pitch (m) I Inertia PS Port side (−) L Lift q Pitch velocity (rad/s) o Fixed coordinate system r Yaw velocity (rad/s) P Regarding the propeller SS Starboard side (−) PS Port side t Time (s) PT Regarding the propeller thrust T Ship draft (m) PTA Oscillation amplitude regarding the propeller TP Thrust in drive bound axis system (N) thrust Txp Longitudinal force in drive bound axis system (N) R Regarding the rudder Typ Lateral force in drive bound axis system (N) SS Starboard side u Longitudinal velocity (m/s) X In longitudinal direction v Lateral velocity (m/s) xx About x-axis w Vertical velocity (m/s) y In lateral direction wR Wake factor (steering) (−) yy About y-axis wT Wake factor (thrust) (−) zz About z-axis V Global velocity in the horizontal plane (m/s) Superscripts x Longitudinal position (m) (eff) Effective X Longitudinal force [derivative of * (* represents (*) (int) Interaction any combination of kinematical parameter(s) u, v, . Derivative w, p, q, r or their derivatives)] (N) Dimensionless x Additional steering induction for N (−) H * Apparent y Lateral position (m) Y(*) Lateral force [derivative of * (* represents any combination of kinematical parameter(s) u, v, w, 1 Introduction p, q, r or their derivatives)] (N) z Vertical position (m) Nowadays more ships tend to be equipped with two shaft Z Vertical force [derivative of * (* represents any (*) lines and two rudders to provide a better manoeuvring per- combination of kinematical parameter(s) u, v, w, formance together with a reduction of emissions to comply p, q, r or their derivatives)] (N) with the EEDI requirements. One of the examples is the z Additional steering induction for K (−) H Maersk Triple E class of container carriers, which started z Steering induction for M (m) HX sailing in 2013. Such vessels offer the possibility to have z Steering induction for Z (−) HZ both engines operated independently, which means that, for Effective inflow angle (°, rad) instance, the portside engine could be turning at dead slow Drift angle (°, rad) ahead, while the starboard engine is working at telegraph Yaw angle (°, rad) position slow. Not only the propellers, but also the rudders Azimuth angle (°, rad) can be operated independently, for instance the portside rud- Hydrodynamic advance angle (°, rad) der could be in its neutral position, while the starboard rud- Δ Displacement (N) der is positioned hard starboard. Such an example is typical Δ∗ Differential (acting on *) (−) for a tug assisted ship, moored along a portside quay, when Pitch angle (°, rad) leaving the harbour. Actual combinations of rudders and/or Regression coefficient (−) propellers depend on various parameters such as the avail- Water density (kg/m3) ability of bow thrusters, tugs, harbour layout and weather Sign (± 1) (−) conditions. Regression coefficient (−) When even a higher level of manoeuvring performance Heel angle; phase angle (°, rad) is required, the steering and propulsion can be joined in one Course angle (°, rad) single device, which is referred to as an azipod, Z-drive or Pulsation (°, rad) L-drive, depending on the layout of the engine. These ves- Subscripts sels have higher turning capabilities as the propulsion jet D Drag is also directed in the way the ship is steered. Two coun- G Regarding centre of gravity ter rotating propellers, which operate in opposite direction, H Regarding the hull mounted on the same drive provide further benefits as they 1 3 Author's personal copy Journal of Marine Science and Technology minimise the unwanted wheel effects. The downside is a open water characteristics and no interaction with the hull is much more complex propulsion and steering system prone investigated. To the authors’ knowledge, only [8, 14] consid- to higher maintenance and incidents. ered characteristics both in open water and mounted behind The vessel discussed in this article is a so-called estuary a flat barge, and [10] implemented an azimuth thruster in a vessel, which is an inland vessel with several adaptations ship-like hull form. However, although the significance of with respect to structural design and equipment to allow their works, the studies consider neither ship–thruster inter- for coastal navigation (estuary navigation) during non-inter- action nor thruster–thruster interaction. This is because units national voyages. Such ships are only allowed to operate were installed outside the hull’s wake, away from each other, under strict conditions regarding draft, freeboard and wave and with the propeller slipstream outside the hull’s bound- height. This is specifically the case in Belgium for trans- ary layer. The analysis of ship–thruster and thruster–thruster port of containers and oil products between the coastal port interactions are required when such devices are installed of Zeebrugge and the port of Antwerp located on the river in an estuary vessel because of the close proximity of the Scheldt, from where the ship can sail further along inland thrusters due to restriction in space, and the overall oper- waterways. The requirements to be met by such vessels are ability which implies a broader range of advance numbers described in a Royal Decree [2]; more background about and azimuth angles. these regulations can be found in [3, 4]. Also in Le Havre (France), inland vessels can be used to connect Port 2000, located at the mouth of the Seine estuary, with the main port, 2 Experimental program as a direct inland waterway connection is missing, if they comply with [5].
Details
-
File Typepdf
-
Upload Time-
-
Content LanguagesEnglish
-
Upload UserAnonymous/Not logged-in
-
File Pages17 Page
-
File Size-