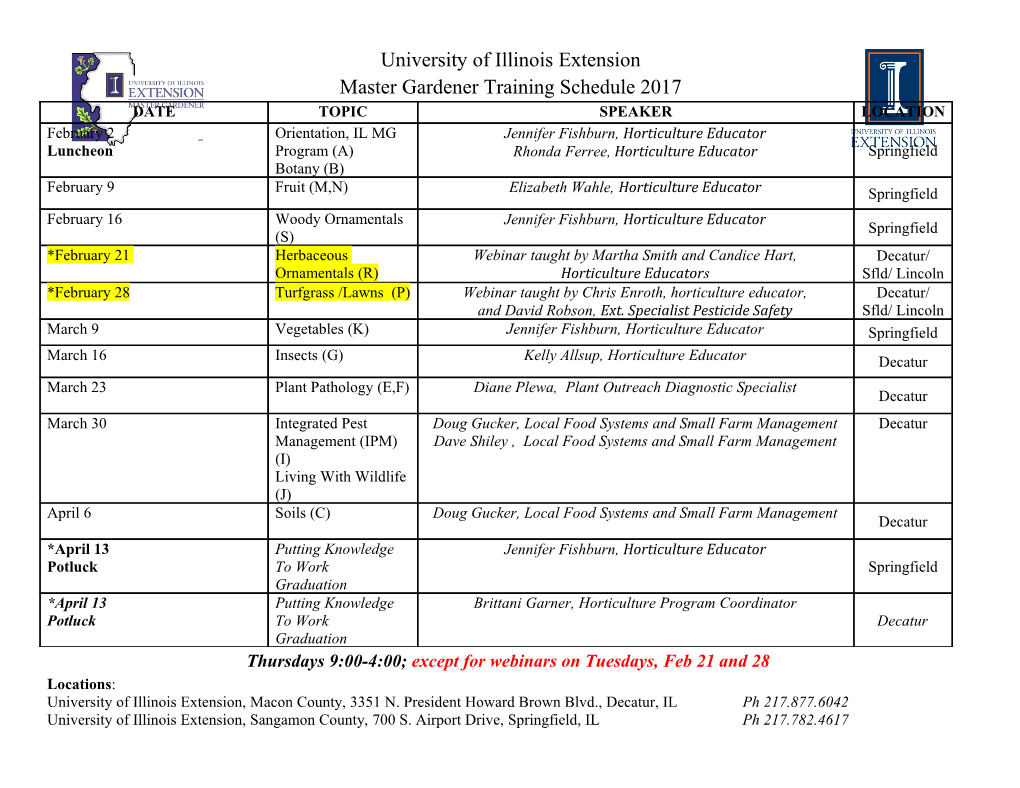
Aquatic Microbiology Single-cell genomics reveals features of a Colwellia species that was dominant during the Deepwater Horizon oil spill Olivia Mason, James Han, Tanja Woyke and Janet Jansson Journal Name: Frontiers in Microbiology ISSN: 1664-302X Article type: Original Research Article Received on: 01 Nov 2013 Accepted on: 16 Jun 2014 Provisional PDF published on: 16 Jun 2014 www.frontiersin.org: www.frontiersin.org Citation: Mason O, Han J, Woyke T and Jansson J(2014) Single-cell genomics reveals features of a Colwellia species that was dominant during the Deepwater Horizon oil spill. Front. Microbiol. 5:332. doi:10.3389/fmicb.2014.00332 /Journal/Abstract.aspx?s=53& /Journal/Abstract.aspx?s=53&name=aquatic%20microbiology& name=aquatic%20microbiology& ART_DOI=10.3389/fmicb.2014.00332 ART_DOI=10.3389 /fmicb.2014.00332: (If clicking on the link doesn't work, try copying and pasting it into your browser.) Copyright statement: © 2014 Mason, Han, Woyke and Jansson. This is an open-access article distributed under the terms of the Creative Commons Attribution License (CC BY). The use, distribution or reproduction in other forums is permitted, provided the original author(s) or licensor are credited and that the original publication in this journal is cited, in accordance with accepted academic practice. No use, distribution or reproduction is permitted which does not comply with these terms. This Provisional PDF corresponds to the article as it appeared upon acceptance, after rigorous peer-review. Fully formatted PDF and full text (HTML) versions will be made available soon. 1 Single-cell genomics reveals features of a Colwellia species that 2 was dominant during the Deepwater Horizon oil spill 3 4 Olivia U. Mason1, James Han2, Tanja Woyke2, and Janet K. Jansson3,4 5 6 1Department of Earth, Ocean and Atmospheric Science, Florida State University, 7 Tallahassee, FL, USA 8 2Department of Energy Joint Genome Institute, Walnut Creek, CA, USA 9 3Ecology Department, Earth Sciences Division, Lawrence Berkeley National Laboratory, 10 Berkeley, CA, USA 11 4Department of Plant and Microbial Biology, University of California, Berkeley, CA 12 13 14 1 15 Abstract 16 17 During the Deepwater Horizon (DWH) oil spill in the Gulf of Mexico a deep-sea 18 hydrocarbon plume developed resulting in a rapid succession of bacteria. Colwellia 19 eventually supplanted Oceanospirillales, which dominated the plume early in the spill. 20 These successional changes may have resulted, in part, from the changing composition 21 and abundance of hydrocarbons over time. Colwellia abundance peaked when gaseous 22 and simple aromatic hydrocarbons increased, yet the metabolic pathway used by 23 Colwellia in hydrocarbon disposition is unknown. Here we used single-cell genomics to 24 gain insights into the genome properties of a Colwellia enriched during the DWH deep- 25 sea plume. A single amplified genome (SAG) of a Colwellia cell isolated from a DWH 26 plume, closely related (avg. 98% 16S rRNA gene similarity) to other plume Colwellia, 27 was sequenced and annotated. The SAG was similar to the sequenced isolate Colwellia 28 psychrerythraea 34H (84% avg. nucleotide identity). Both had genes for denitrification, 29 chemotaxis and motility, adaptations to cold environments and a suite of nutrient 30 acquisition genes. The Colwellia SAG may be capable of gaseous and aromatic 31 hydrocarbon degradation, which contrasts with a DWH plume Oceanospirillales SAG 32 genome which encoded non-gaseous n-alkane and cycloalkane degradation. The 33 disparate hydrocarbon degradation pathways are consistent with hydrocarbons that were 34 abundant at different times in the deep-sea plume; first, non-gaseous n-alkanes and 35 cycloalkanes that could be degraded by Oceanospirillales, followed by gaseous, and 36 simple aromatic hydrocarbons that may have been degraded by Colwellia. These insights 37 into the genomic properties of a Colwellia species, which were supported by existing 38 metagenomic sequence data from the plume and DWH contaminated sediments, help 39 further our understanding of the successional changes in the dominant microbial players 40 in the plume over the course of the DWH spill. 41 2 42 Introduction 43 44 The Deepwater Horizon (DWH) oil spill from April to July 2010 was unprecedented due 45 to the extreme depth (1500 meters below sea-level; mbsl) and low temperature (4°C), at 46 which it took place. Colwellia species bloomed in the deep-sea hydrocarbon plume that 47 formed at 1100 mbsl in early June, 2010 (Valentine et al. 2010; Redmond & Valentine 48 2012) after partial capture of the oil began (Dubinsky et al. 2013). At this time the 49 unmitigated flow of oil ceased, and cycloalkanes and non-gaseous n-alkanes, which were 50 dominant until that point, decreased in concentration (Dubinsky et al. 2013). 51 Concomitantly, the concentration of natural gases and simple aromatics increased 52 (Dubinsky et al. 2013). The change in hydrocarbon composition and abundance resulting 53 from partial capture was mirrored by differences in microbial community composition, 54 with a shift in dominance of Oceanospirillales to Colwellia (Dubinsky et al. 2013). 55 Mason et al. (2012) analyzed Oceanospirillales single amplified genomes (SAGs) from 56 the deep-sea plume and reported the genome encoded pathways for cyclohexane and non- 57 gaseous n-alkane degradation. These data helped to explain the dominance of 58 Oceanospirillales at the time when cycloalkanes and non-gaseous n-alkanes, and its 59 subsequent decline when these hydrocarbon constituents were less abundant. The later 60 appearance of Colwellia in the spill history could be due to its ability to degrade other 61 hydrocarbon constituents in the oil. Microcosm experiments established that Colwellia 62 species were capable of degrading hydrocarbons originating from the oil spill during 63 incubations at 4°C (Bælum et al. 2012; Redmond & Valentine 2012). Specifically, 64 Redmond & Valentine (2012) reported that Colwellia species from the deep-sea plume 65 incorporated labelled ethane, propane and benzene. The ability to degrade ethane and 66 propane, for example, provides clues as to why Colwellia appears to have increased in 67 abundance when the concentration of these and other gases increased in June 2010. 68 69 Hydrocarbon degradation by cultured Colwellia has not previously been reported (e.g. 70 Methé et al. 2005; Bowman et al. 1997). For example, Colwellia psychrerythraea 34H 71 that was cultivated from Arctic marine sediments, is psychrophilic, with optimal growth 72 at -1°C to 10°C (Huston 2003) but it was not reported to degrade hydrocarbons. The 73 genome of C. psychrerythraea provides insights into the adaptations that enable it to be 74 active at such low temperatures, such as changes to cell membrane fluidity and the use of 75 compounds that provide cryotolerance (Methé et al. 2005). Further, this genome 76 provides a platform for comparison to Colwellia that were enriched during the DWH oil 77 spill. Although the possible role of C. psychrerythraea in bioremediation by aromatic, or 78 C1 contaminant degradation was inferred, the specific contaminants and the pathways 79 catalyzing these reactions have not yet been elucidated (Methé et al. 2005). Thus it 80 remains unresolved as to how Colwellia species that were identified during the DWH 81 spill are capable of growth with ethane, propane and benzene, (Redmond & Valentine 82 2012), polycyclic aromatic hydrocarbons (PAH) (Gutierrez et al. 2013), or MC252 crude 83 oil constituents (Bælum et al. 2012). Here our aim was to use single-cell genomics to 84 gain a better understanding of the genomic properties of a deep-sea Colwellia species that 85 enabled it to bloom during the oil spill. Specifically, we present a genome analysis of a 86 Colwellia single-cell isolated directly from the deep-sea plume described in Hazen et al 87 (2010) and Mason et al. (2012). 3 88 89 Materials and Methods 90 Single-cell sorting, whole-genome amplification and screening 91 Cells were collected following the clean sorting procedures detailed by Rodrigue et al. 92 (2009). Briefly, single cells from the proximal plume water sample, collected on May 29, 93 2010 from 1207 mbsl (described in Mason et al. (2012)), were sorted by the Cytopeia 94 Influx Cell Sorter (BD Biosciences, Franklin Lakes, NJ) into three 96-well plates 95 containing 3 µl of ultraviolet-treated TE. The cells were stained with SYBR Green I 96 (Invitrogen, Carlsbad, CA) and illuminated by a 488-nm laser (Coherent Inc., Santa 97 Clara, CA). As described by Woyke et al. (2011) the sorting window was based on the 98 size determined by side scatter and green fluorescence (531/40 bp filter). Single cells 99 were lysed for 20 min at room temperature using alkaline solution from the Repli-G 100 UltraFast Mini Kit (Qiagen, Valencia, CA) according to the manufacturer's instructions. 101 After neutralization, the samples were amplified using the RepliPHI Phi29 reagents 102 (Epicentre Biotechnologies, Madison, WI). Each 50-µl reaction contained Phi29 Reaction 103 Buffer (1 × final concentration), 50 µM random hexamers with the phosphorothioate 104 bonds between the last two nucleotides at the 3′ end (IDT, Coralville, IA), 0.4 mM dNTP, 105 5% DMSO (Sigma, St Louis, MO), 10 mM DTT (Sigma), 100 U Phi29 and 0.5 mM Syto 106 13 (Invitrogen). A mastermix of multiple displacement amplification (MDA) reagents 107 minus the Syto 13 sufficient for a 96-well plate was ultraviolet-treated for 60 min for 108 decontamination. Syto 13 was then added to the mastermix, which was added to the 109 single cells for real-time MDA on the Roche LightCycler 480 for 17 h at 30 °C. All steps 110 of single-cell handling and amplification were performed under most stringent conditions 111 to reduce the introduction of contamination. Single-cell MDA products were screened 112 using Sanger sequencing of 16S rRNA gene amplicons derived from each MDA product.
Details
-
File Typepdf
-
Upload Time-
-
Content LanguagesEnglish
-
Upload UserAnonymous/Not logged-in
-
File Pages21 Page
-
File Size-