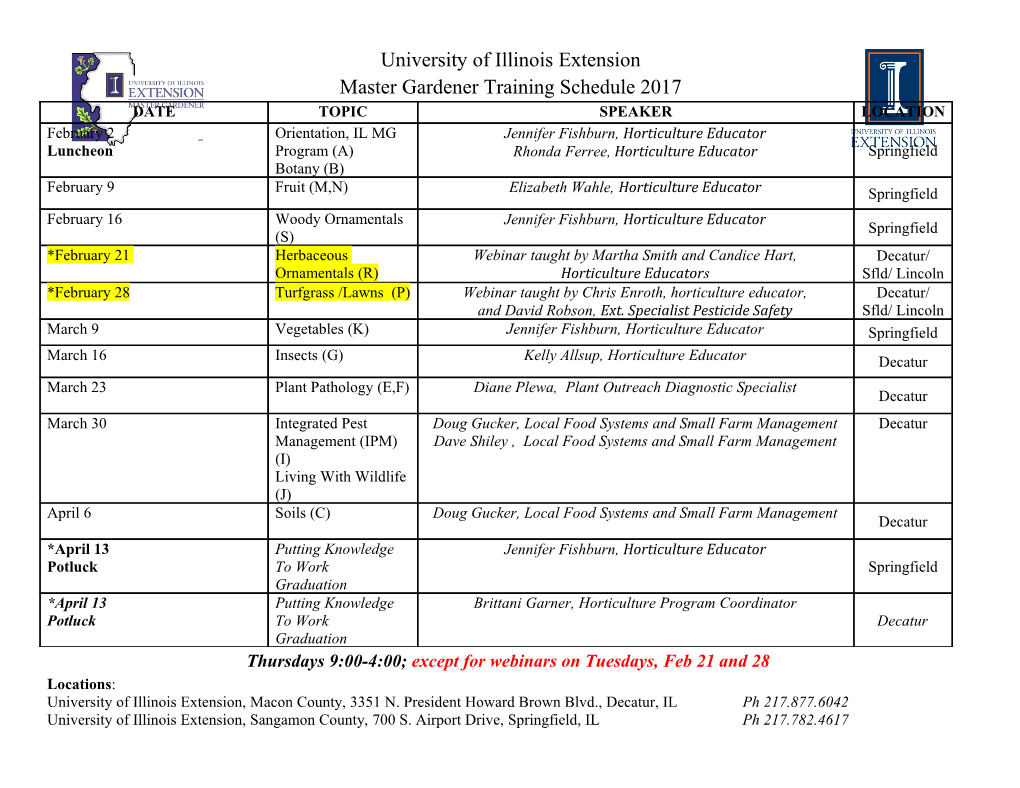
Chapter 6 Protein Structure and Folding 1. Secondary Structure 2. Tertiary Structure 3. Quaternary Structure and Symmetry 4. Protein Stability 5. Protein Folding Myoglobin Introduction 1. Proteins were long thought to be colloids of random structure 2. 1934, crystal of pepsin in X-ray beam produces discrete diffraction pattern -> atoms are ordered 3. 1958 first X-ray structure solved, sperm whale myoglobin, no structural regularity observed 4. Today, approx 50’000 structures solved => remarkable degree of structural regularity observed Hierarchy of Structural Layers 1. Primary structure: amino acid sequence 2. Secondary structure: local arrangement of peptide backbone 3. Tertiary structure: three dimensional arrangement of all atoms, peptide backbone and amino acid side chains 4. Quaternary structure: spatial arrangement of subunits 1) Secondary Structure A) The planar peptide group limits polypeptide conformations The peptide group ha a rigid, planar structure as a consequence of resonance interactions that give the peptide bond ~40% double bond character The trans peptide group The peptide group assumes the trans conformation 8 kJ/mol mire stable than cis Except Pro, followed by cis in 10% Torsion angles between peptide groups describe polypeptide chain conformations The backbone is a chain of planar peptide groups The conformation of the backbone can be described by the torsion angles (dihedral angles, rotation angles) around the Cα-N (Φ) and the Cα-C bond (Ψ) Defined as 180° when extended (as shown) + = clockwise, seen from Cα Not all combinations of torsion angles are possible, some result in collision of N-H with O=C or R The Ramachandran diagram indicates allowed conformations of polypeptides • Pro limits Φ to -60° • Gly allows more freedom B) The most common regular secondary structures are the α Helix and the β Sheet • Two structures are widespread: α Helix and β Sheet = regular secondary structures • The α helix is a coil: structure with allowed angles and stabilized by hydrogen bonding • Linus Pauling, 1951, model building • Right-handed turn • Pitch 3.6 Aa/turn = 5.4 Å • Average length 12 Aa = 3 turns ~18 Å • Bonding of C=O with N-H at n+4 • R point outwards, downwards β sheets are formed from extended chains • like α helix, β sheets are stabilized by hydrogen bonding • Bonding occurs between neighboring chains rather than within the structure as is the case with α helix • Parallel and antiparallel Pleated appearance of a β sheet • From 2 to 22 strands • Average 6 strands in protein • Exhibit right-handed twist 7-stranded Bovine carboxypeptidase A Coils Helices β-sheet Turns connect some units of secondary structure • topology = connectivity of β sheets can be complex or made by a simple turn/loop • reverse turns or β bends, occur at the protein surface, connect β sheets Reverse turns in polypeptide chains • Require 4 Aa • 180° flip of Aa 2 C) Fibrous proteins have repeating secondary structure Historical classification of proteins as globular of fibrous Fibrous proteins often have protective, connective, or supportive role in living organisms examples: keratin and collagen α keratin is a coiled coil Keratin, in all higher vertebrates - horny outer epidermal layer - hair, horn, nails, feathers - α in mammals - β in birds and reptiles - Humans, over 50 α genes - tissue specific expression Forms coiled coil structure - helix pitch 5.1 Å (rather than 5.4 Å as in α-helix) - Parallel N->C, 81 residues long Coiled coil has a pseudo-repeating structure: a-b-c-d-e-f-g in which residues a and d are predominately nonpolar. Helical wheel representation Higher order α keratin structure -> Macrofibril -> single hair α keratin α keratin is rich in Cys residues, form disulfide bonds that crosslink adjacent chains α keratins are classified “hard” and “soft” depending on whether they have high or low sulfur content Hard keratins in hair, horn, and nails are less pliable than soft keratins such as in skin and callus (hornhaut) Reductive cleavage of disulfide bonds results in “curled hairs” Collagen is a Triple Helix Collagen occurs in all multicellular organism, - Is the most abundant vertebrate protein - Strong insoluble fibers - Stress bearing component of connective tissues (bone, teeth, cartilage, tendon) One collagen molecule consists of three polypeptide chains - Mammals have at least 33 genetically distinct chains - Assembled in 20 different collagen varieties - One of the most common is Type I collagen: - two α1(I) chains and one α2(I) chain Collagen has a distinctive amino acid composition: - 1/3 of its residues is Gly - 15 to 30% are Pro and 4-hydroxyprolyl (Hyp) These non-standard amino acids are formed after collagen is synthesized - Pro - > Hyp conversion by prolyl hydroxylase, Vitamin C-dependent (ascorbic acid) Collagen Diseases Scurvy (Skorbut) results from Vitamin C-deficiency, - Lack of newly synthesized functional collagen, - Skin lesion, fragile blood vessels, poor wound healing - Sailors on long trips, lack of fresh food - Captain James Cook “limely” introduced limes to diet Several rare genetic collagen disorders - tend to be dominant The Collagen Triple Helix Collagen amino acid sequence consists of a repeating triplet: Gly-X-Y over some 1000 residues, X often Pro, Y often Hyp - Pro prevents formation of α helix - Collagen forms left-handed superhelix with ~3 amino acids per turn - Three parallel chains wind around each other to form right-handed triple-helical structure - Every third residue of each chain passes through the center if the triple helix, only Gly can pass - Three chains are staggered, Gly at each level - Inter-chain hydrogen bonding Structure of collagen model peptide Hydrogen bonding from Gly N- to Pro O-atoms of adjacent chain Collagen Crosslinking Collagens well-packed, rigid, triple-helical structure is responsible for its characteristic tensile strength Collagens assemble to form loose networks or thick fibrils arranged in bundles or sheets, depending on the tissue Collagen is internally covalently cross linked which makes it insoluble, not by Cys but by Lys and His reactions Lysyl oxidase reaction: D) Most proteins include nonrepetitive structures Majority of proteins are globular and may contain several types of regular secondary structures, including α helices and β sheets and coils (≠ random coils, which are completely unstructured) The sequence affects the secondary structure: - Pro is helix breaking, induces kink in helix and β sheet - Large amino acids such as Tyr and Ile induce steric clashes - Peter Chou and Gerald Fasman revealed the propensity of amino acids to form helical or sheet structures -> Chou-Fasman prediction 2) Tertiary Structure Tertiary structure of a protein describes the folding of its secondary structural elements and specifies the position of every atom in the protein This information is deposited in protein structure database (pdb) Experimentally determined by X-ray crystallography or NMR Protein crystals A) Most proteins structures are determined by X- ray crystallography or nuclear magnetic resonance X-Ray crystallography: technique that directly images molecules - X-ray wave length is short, ~1.5 Å, equivalent to distance of atoms (visible light 4000 Å) - Crystal: repetitive arrangement of the same structure => diffraction pattern (darkness of spot is function of crystals electron density) - X-ray interact with electrons (not with nuclei) -> X-ray structure is thus an electron density map of a given protein -> represents contours of atoms A thin section through a 1.5 Å resolution electron density map of a protein that is contoured in three dimensions Most protein crystal structures exhibit less than atomic resolution Crystal is build up by repeating units, containing protein in native conformation, - highly hydrated (40-60% water) - soft, jellylike consistency, unlike NaCl crystals - molecules are slightly disordered and display Brownian motion -> this determines the resolution limit of a given protein crystal (typical 1.5 – 3 Å) - inability to crystallize a protein to form crystals of sufficiently high resolution is a major limiting factor in structure determination Electron density maps of diketopiperazine at different resolution levels - Electron density map alone is not sufficient to determine the structure if the protein, - Amino acid sequence is also required - Computerized fitting algorithm of atoms into the experimentally determined electron density map results in protein structure determination of up to 0.1 Å resolution Most crystallized proteins maintain their native conformations Key question: does the structure of protein in a crystal accurately reflect the structure of the protein in solution, where it normally functions ? 1. The protein in the crystal is hydrated like it is in solution 2. X-ray structure is similar to NMR structure, which is determined from proteins that are in solution 3. Many enzymes remain catalytically active in the crytsal Protein structures can be determined by NMR Nuclear magnetic resonance, NMR, an atom nucleus resonates if a magnetic field is applied. This resonance is sensitive to the electronic environment of the nucleus and its interaction with nearby nuclei - Developed since 1980, Kurt Wüthrich (ETH-Z), to determine protein structures in solution - Because there are many nuclei in a protein that would crowd in a conventional one-dimensional NMR -> two- dimensional (2D) NMR was developed to measure atomic distances of chemically linked atoms (COSY) or of spatially close atoms (NOESY) - Size limit of about 40 kD, may reach 100 kD soon - Dynamic, can follow protein motion or folding B) Side chain location varies with polarity Since Kendrew solved the first protein structure, nearly 50’000 protein structures have been reported No two are the same, but they exhibit some remarkable consistency: globular structures lack the repeating sequences that support the conformation of fibrous proteins Amino acid side chains in globular proteins are distributed according to their polarity: 1. Nonpolar residues Val, Leu, Ile, Met and Phe occur mostly in the interior of a protein, excluded from the contact with water, hydrophobic core, compact packing (no empty room) 2.
Details
-
File Typepdf
-
Upload Time-
-
Content LanguagesEnglish
-
Upload UserAnonymous/Not logged-in
-
File Pages109 Page
-
File Size-