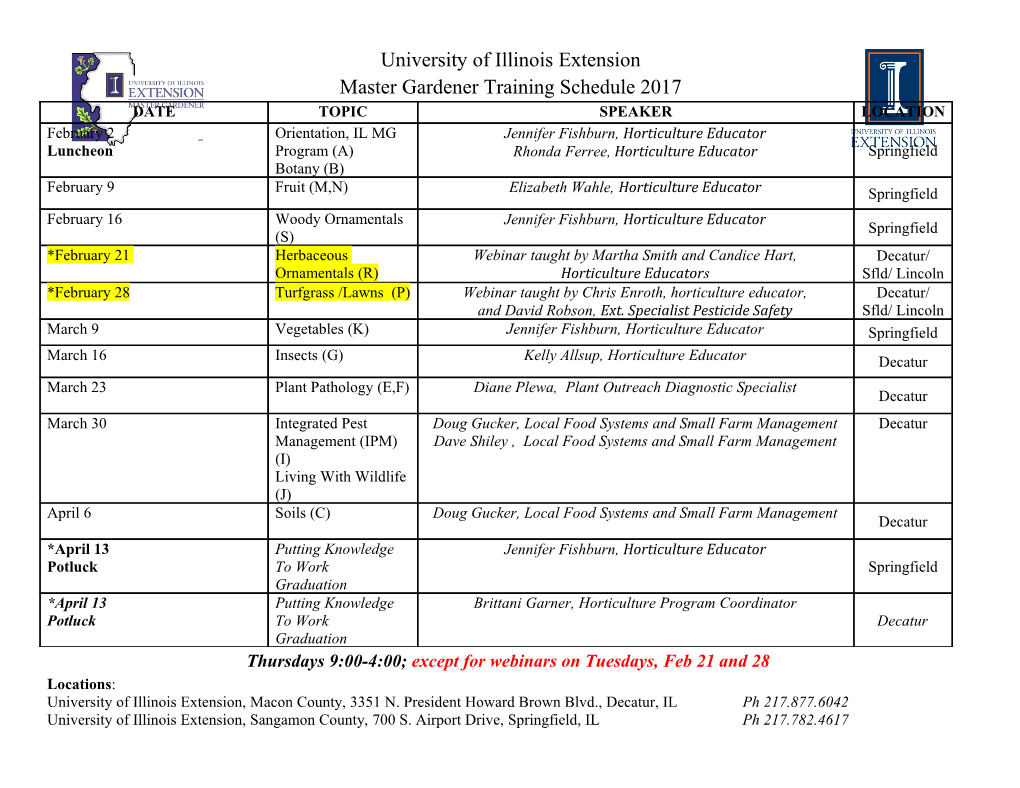
Nuclear Astrophysics: The origin of heavy elements Lecture 3: The r process Gabriel Martínez Pinedo Isolde lectures on nuclear astrophysics May 9–11, 2017 Signatures of heavy element nucleosynthesis Nucleosynthesis in core-collapse supernovae Nucleosynthesis in neutron star mergers Summary Outline 1 Signatures of heavy element nucleosynthesis 2 Nucleosynthesis in core-collapse supernovae 3 Nucleosynthesis in neutron star mergers Dynamical ejecta Accretion disk ejecta 4 Summary Signatures of heavy element nucleosynthesis Nucleosynthesis in core-collapse supernovae Nucleosynthesis in neutron star mergers Summary Nucleosynthesis beyond iron Three processes contribute to the nucleosynthesis beyond iron: s process, r process and p process (γ-process). 10 2 80 1 10 s r s ] 0 70 p-drip 6 10 r 10 -1 60 -2 Z 10 50 10 -3 115 Sn 180 p n-drip -4 138 W 40 10 La Abundances [Si=10 152 10 -5 Gd 30 164 180 m Ta 10 -6 Er 40 60 80 100 120 80 100 120 140 160 180 200 N A 10−12 −3 s process: low neutron densities, nn = 10 cm , τn > τβ (site: intermediate mass stars) 20 −3 r process: large neutron densities, nn > 10 cm , τn τβ (unknown astrophysical site) p process: photodissociation of s-process material. Signatures of heavy element nucleosynthesis Nucleosynthesis in core-collapse supernovae Nucleosynthesis in neutron star mergers Summary Time evolution: metalicity [Fe/H] ~ -5 [Fe/H] ~ -3 Astronomers use the metalicity: ! ! NFe NFe [Fe=H] = log10 − log10 NH ∗ NH as a proxy for age. Signatures of heavy element nucleosynthesis Nucleosynthesis in core-collapse supernovae Nucleosynthesis in neutron star mergers Summary Evolution metalicity Sneden, Cowan & Gallino 2008 a 1.5 1.0 Core-collapse Supernovae Type Ia 0.5 [Mg/Fe] 0 – 0.5 b 1.5 1.0 0.5 [Eu/Fe] 0 – 0.5 –3 –2 –1 0 [Fe/H] r process occurs already at early galactic history Large scatter at low metalicities: r process is related to rare events not correlated with iron. Signatures of heavy element nucleosynthesis Nucleosynthesis in core-collapse supernovae Nucleosynthesis in neutron star mergers Summary Heavy elements and metal-poor stars Cowan & Sneden, Nature 440, 1151 (2006) Stars rich in heavy r-process elements (Z > 50) 0 and poor in iron (r-II stars, [Eu=Fe] > 1:0). ε Robust abundance patter for Z > 50, −2 consistent with solar r-process abundance. −4 Abundances are the result of events that do Relative log not produce iron. [Qian & Wasserburg, Phys. −6 Rept. 442, 237 (2007)] −8 30 40 50 60 70 80 90 Atomic Number 0.5 (b) Sr 0 Zr translated pattern of CS 22892-052 (Sneden et al. 2003) Ru -0.5 Mo Pd -1 Stars poor in heavy r-process elements but ) Y Z ( ε -1.5 with large abundances of light r-process Nb log -2 elements (Sr, Y, Zr) Ag -2.5 Production of light and heavy r-process -3 HD 122563 (Honda et al. 2006) Eu elements is decoupled. -3.5 40 50 60 70 80 Atomic Number (Z) Honda et al, ApJ 643, 1180 (2006) Signatures of heavy element nucleosynthesis Nucleosynthesis in core-collapse supernovae Nucleosynthesis in neutron star mergers Summary r-process astrophysical sites Core-collapse supernovae Neutron star mergers Explosion of massive stars Mergers eject around 0:01 M of very (M & 9 M ) neutron rich-material (Ye ∼ 0:01). Similar amount of less neutron-rich matter Site: neutrino-winds from cooling (Y 0:2) ejected from accretion disk. of hot protoneutron star. e & Low frequency, high yield High frequency (∼ 0:3 yr−1), low −4 −5 yield ejecta (10 –10 M ) Observational signature: electromagnetic transient from radioactive decay of Observations: not every r-process nuclei [Kilonova/Macronova, supernovae produces r process Metzger et al (2010)] Signatures of heavy element nucleosynthesis Nucleosynthesis in core-collapse supernovae Nucleosynthesis in neutron star mergers Summary Measurements long-lived radioactive nuclei Both the 244Pu (τ = 81 Myr) accreted via interstellar particles in the Earth deepsea floor and the Early Solar System abundanced are naturally explained with the low-rate/high-yield astrophysical scenario. [Hotokezaka et al, Nature Physics 11, 1042 (2015)] CC Supernova 10,000 LIGO/Virgo R-element mass ( Macronova 1,000 candidate ) 1 A − Pu ≥ r 90) 100 My ( 0 R 10 Compact binary merger 1 Advanced LIGO/ Virgo/KAGRA 0.1 0.0001 0.001 0.01 0.1 1 Mej (Msun) Any astrophysical site should fulfill: RMej = M(A > 90) Signatures of heavy element nucleosynthesis Nucleosynthesis in core-collapse supernovae Nucleosynthesis in neutron star mergers Summary Core-collapse supernovae H.-Th. Janka, et al, PTEP 01A309 (2012) " ! The movie of the supernova simulation is available here Signatures of heavy element nucleosynthesis Nucleosynthesis in core-collapse supernovae Nucleosynthesis in neutron star mergers Summary Neutrino-driven winds Main processes: − νe + n p + e + ν¯e + p n + e Neutrino interactions determine the proton to neutron ratio. Neutrino cooling and Neutrino-driven wind Condition neutron-rich ejecta: 105 Lν¯e h i hEν¯ i − hEν i > 4∆np − − 1 hEν¯ i − 2∆np e e Lν e ν , ν e 104 e,µ,τ e,µ,τ m in k R Ni neutron-rich ejecta: r-process 103 Si He proton-rich ejecta: νp-process 102 νp-process ν , –ν r-process O e,µ,τ e,µ,τ R Nucleosynthesis sensitive to spectral ns ~10 Rν PNS differences between ν and ν¯ 1.4 α, p α, p, nuclei 3 e e M(r) in M n, p α, n α, n, nuclei Signatures of heavy element nucleosynthesis Nucleosynthesis in core-collapse supernovae Nucleosynthesis in neutron star mergers Summary Evolution luminosities and Ye 1D Boltzmann transport radiation simulations (artificially induced explosion) for a 11.2 M progenitor based on the DD2 EoS (Stefan Typel and Matthias Hempel). 1052 0.60 0.58 0.56 51 0.54 10 0.52 0.50 0.48 50 Electron fraction 0.46 Luminosity [ergs/s] 10 0 2 4 6 8 10 0 2 4 6 8 10 Time [s] Time [s] 13 100 12 νe 90 11 80 ν¯e /baryon] 70 10 B [MeV] k 60 i 9 νx ν 50 E 8 40 h 7 30 6 20 0 2 4 6 8 10 Entropy [ 0 2 4 6 8 10 Time [s] Time [s] Ye is moderately neutron-rich at early times and later becomes proton-rich. GMP, Fischer, Huther, J. Phys. G 41, 044008 (2014). Signatures of heavy element nucleosynthesis Nucleosynthesis in core-collapse supernovae Nucleosynthesis in neutron star mergers Summary Nucleosynthesis 107 6 10 11.2 105 104 103 102 101 100 1 10− 2 Rel. abundance 10− 3 10− 4 10− 50 60 70 80 90 100 110 Mass number A 2 10− 3 10− HD 122563 4 10− 5 10− 6 10− 7 10− 8 10− Elemental abundance 9 10− 25 30 35 40 45 50 55 Charge number Z Elements between Zn and Mo (A ∼ 90) are produced Mainly neutron-deficient isotopes are produced Signatures of heavy element nucleosynthesis Nucleosynthesis in core-collapse supernovae Nucleosynthesis in neutron star mergers Summary Compact binary systems: gravitational waves Orbital decay Hulse & Taylor binary pulsar Gravitational waves binary black holes merger First detection! 9:50:45 UTC, 14 September 2015 LIGO Hanford signal LIGO Livingston signal Abbott et al, PRL 116, 061102 (2016) Weisberg & Huang, ApJ 829, 55 (2016) Compact binary systems exist and eventually merge Signatures of heavy element nucleosynthesis Nucleosynthesis in core-collapse supernovae Nucleosynthesis in neutron star mergers Summary Merger channels and ejection mechanism In mergers we deal with a variety of initial configurations (netron-star neutron-star vs neutron-star black-hole) with additional variations in the mass-ratio. The evolution after the merger also allows for further variations. NSNS wind; t~100 ms torus unbinding; dyn. ejecta; t~ 1 ms t ~ 1s ⇒ ⇒ ⇒ ⇒ ⇒ ⇒ ⇒ BH formation ⇒ sGRB ⇒ ⇒ ⇒ ⇒ ⇒ ⇒ ⇒ NSBH Rosswog, et al, arXiv:1611.09822 Signatures of heavy element nucleosynthesis Nucleosynthesis in core-collapse supernovae Nucleosynthesis in neutron star mergers Summary Making Gold in Nature: r-process nucleosynthesis 250 Known mass Known half−life r−process waiting point (ETFSI−Q) 100 200 98 96 94 92 90 88 Solar r abundances 86 84 188 190 186 82 80 78 184 180 182 76 178 176 74 164 166 168 170 172 174 150 72 162 70 160 N=184 68 158 66 156 154 64 152 150 62 140 142 144 146 148 1 60 138 134 136 58 130 132 0 128 10 56 54 126 124 10 −1 52 122 The r-process requires the knowledge of 120 50 116 118 10 −2 48 112 114 110 46 −3 108 10 100 106 N=126 44 104 the properties of extremely 100 102 42 10 98 96 40 92 94 38 88 90 86 84 r−process path 36 82 neutron-rich nuclei: 34 80 78 32 74 76 30 72 70 28 66 68 64 26 62 N=82 28 60 30 32 34 36 38 40 42 44 46 48 50 52 54 56 58 Nuclear masses. Beta-decay half-lives. Neutron capture rates. Fission rates and yields. Signatures of heavy element nucleosynthesis Nucleosynthesis in core-collapse supernovae Nucleosynthesis in neutron star mergers Summary Evolution nucleosynthesis in mergers 528 trajectories r-process stars once electron fermi energy drops below ∼ 10 MeV to allow for beta-decays (ρ ∼ 1011 g cm−3). Important role of nuclear energy production (mainly beta decay). Energy production increases temperature to values that allow for an (n; γ) (γ; n) equilibrium for most of the trajectories. Systematic uncertainties due to variations of astrophysical conditions and nuclear input Mendoza-Temis, Wu, Langanke, GMP, Bauswein, Janka, PRC 92, 055805 (2015) Signatures of heavy element nucleosynthesis Nucleosynthesis in core-collapse supernovae Nucleosynthesis in neutron star mergers Summary Final abundances different mass models Mendoza-Temis, Wu, Langanke, GMP, Bauswein, Janka, PRC 92, 055805 (2015) FRDM WS3 10-3 10-4 10-5 10-6 abundances at 1 Gyr 10-7 10-8 HFB21 DZ31 10-3 10-4 10-5 10-6 abundances at 1 Gyr 10-7 10-8 120 140 160 180 200 220 240 120 140 160 180 200 220 240 mass number, A mass number, A Robustness astrophysical conditions, strong sensitivity to nuclear physics Second peak (A ∼ 130) sensitive to fission yields.
Details
-
File Typepdf
-
Upload Time-
-
Content LanguagesEnglish
-
Upload UserAnonymous/Not logged-in
-
File Pages28 Page
-
File Size-