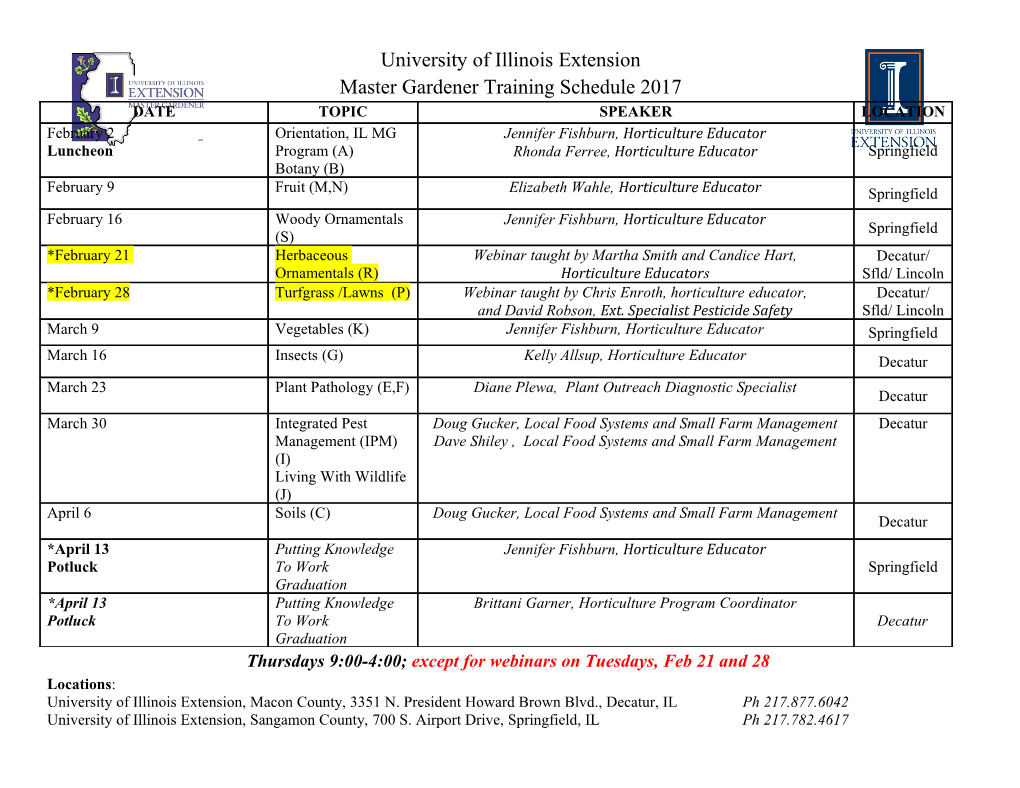
r , ELECTROLYTIC PRODUCTION OF ALUMINIUM:� FUNDAMENTAL AND APPLIED STUDIES ON THE EFFECT OF ANODE COMPOSITION ON TEE ANODE-ELECTROLYTE INTERFACIAL CONDITIONS. by :,:.!::_OSBORNE. B.Sc. (Hons.), (Tas.) being a Thesis submitted. in fulfilment of the require­ ments for the Degree of Master of Science UNIVERSITY OF TASMANIA HOBART'. (May 1968) DECLARATION OF ORIGINALITY All of the experimental w0rk in this thesis, except where directly ascribed to others, has been performed solely by the auth0r. No part 0f the text bas previoµsly been submitted f@r any other degree or diplGma in any University, nor dees it contain, to the best knowledge of the undersigned, any material which has been published by others, except where due reference bas been SQ made. SUMMARY This thesis deals with a study of the wettability of amorphous carbon, of the type empl©yed as anode material in the production of aluminium, by cryolite­ based solutions. The wettability of carbon has been improved by the addition of inorganic additives 0f the type used in cryolite electrolytes, such as aluminium oxide, calcium flanride and carbonate, and lithium flunride and carbonate. Particular improvements in carbon wettability are noted when the system is contained in an atmosphere of carbon dioxide - carbon monoxide, corresponding to practical production conditions. Furtheremore, when anodes prepared c0ntaining these additives are used in a laboratory aluminium cell, notable reductions in cell voltage are achieved at practical an0de current densities. The improved adhesion of the two phases has also been demonstrated by measurement of the current density and voltage con­ ditions required to llinduce anode effects in this cell •. Improvements in 110wer requirements and carb©n consumption per unit weight of product are shown, and finally, an assessment of the project potential under industrial operating conditions has been made. TABLE O'F CONTENTS SUMMARY INTRODUCTION PART ]: CONTACT ANGLE STUDIES. (a) General (b) Experimental (i) Anode Preparation (ii) Contact Angle Measurements. (c) Results (i) Anode Preparation and Properties (ii) Contact Angle Furnace Operation (iii) Observation of Melting Phenomena (iv) Contact Angle Measurements~ (d) Discussion PART II: LABORATORY REDUCTION CELL STUDIES (a) General (b) Melt Structure ( c) Experimental (i) Construction of Cell (ii) Raw materials for Cell (iii) Cell 0-peration (d) Results (i) Operation of Reduction Cell (ii) Current - Voltage Measurements Table of Contents·continued. (d) Results cont. (iii) Critical Current Density Measurements (iv) Current Efficiency and Carbon Consumption. (e) Discussion PART III: CONCLUSION (a) Preliminary Plant Measurements (b) Industrial Implementation ACKNOWLEDGEMENTS REFERENCES' APPENDIX A: ANALYTICAL PROCEDURES APPENDIX B: MISCELLANEOUS ANODE PROPERTIES 1. INTRODUCTION~ The work described herein was under.taken primariJLy as an applied industrial study at the Comalco Aluminium (Bell Bay) Limited aluminium smelter. The overall object was the lowering of the individual reduction cell voltage as a means of improving the energy effic­ iency of the Hall-Heroult electrolytic process for the manufacture of aluminium and its alloys. It is well known in the aluminium industry that the electrolysis voltage required to deposit aluminium from a cryolite-based electrolyte is in practice of the order of 4 to 5 volts. This compares with theoretical values of between 1.01v and 2.22V (1) depending on the type of electrodes and overall reactions that are assumed. The following theoretical reversible decomposition potentials at 1000°c with the reactants in their stan­ dard states have been found; 1.01V for the reaction A~203 '* 3C = 2Al + 3CO (Ref. 1 ) ' and 1.16v for the reaction Al20i3 + ~c -= 2Al + ~co ;t. (Ref. 1);- from thermodynamic data, we have 2.22V for the reaction Al203 (s) = 2Al(l) + ~Ol2(g) (Ref. 1 ). In practice the decomposition voltage lies between 1.45 and 1.65V, the overvoltage being mainly anodic (2). 2. While a certain amount of the excess energy, at the high currents used industrially, is necessary to maintain the electrolyte in a molten condition at its operating temperature (of the order of 980°c), it can be seen, as various workers (3,4,5) have shown, that a significant factor in the energy requirements is the anode overvoltage. Alth0ugh conflicting explanations exist as to the mechanism of this overvoltage, typical figures at practical curnnt densities, alumina concen­ trations, and cell temperatures are of the order of e.4 - o.7v. In an aluminium reduction cell the operating pro­ cess normally consists of the continuous downward feeding of a carbonaceous anode, comprising a petroleum/ coke/pitch mixture, into a molten bath of a mixture of cryolite, aluminium oxide, and calcium fluoride. This molten electrolyte is contained-in a carbon-lined stee~ shell, which acts as the cathode. At the anode, the tw~ surfaces directly in contact with each other are the carbon electrode and the cryolitic electrolyte. Structurally, these two mat­ erials are profoundly dissimilar, the former consisting of a covalent solid, with a varying degree of graphitic structure, and the latter consisting of an ionic melt. One result of this structural difference is a marked lack of physical adhesion or wettability under certain 3. atmospheric environments (6), which manifests itself in an imperfect electrical contact. This contributes to a higher voltage than is desirable under plant operating conditions. In addition to the 0verv0ltage pr0duced in an operating cell, the slow release of the primary anode product, co 2, allows the formation of significant am0unts of carbon monoxide, resulting in a carbon usage of approximately 0.5 lb. for every one pound of aluminium ,produced, c0mpared with a theoretical usage of 0.33 lb. of carbon fer the reaction:- 2Al203 + 3C = 4A]_ + 3co2 Therefore if impr0ved physical and electrical contact can be established between the two interfacial surfaces, a lower total voltage drop per cell can be expected. Also, if a m0re rapid release of anode gases can be realised, a reduction in carbon consumption can be expected. The experimental route chosen for this project has been to study the effect of incorporating small amounts of inorganic additives to the unbaked carbon mixture. These additives have been chosen to promote the contact between anode and electrolyte. Because of the applied nature of this work, the choice of additive materials bas been restricted to those which are compatible with the normal industrial production of aluminium. Such 4. compounds include aluminium oxide, calcium fluoride, calcium carbonate, aluminium fluoride, and certain lithium compounds. The study of the use of these compounds is described in the subsequent two maj0r sections:- (!) Contact Angle Studies (ii) Laboratory Reduction Cell Studies. From the point of view of both local application and experimental technique, only baked anodes were studied. Although similar additives could be made to Soderberg electrodes, this aspect was n0t investigated, as such, in this work. P~R~' I .• CONTACT ANGLE STUiDii,& (a) General: When a drop of liquid rests under equilibrium on a horizontal solid surface, a configuration of the following type is adopted:- Vapour Liquid In such a system, the interfacial surface tensions are related by the following expression:- "5SV ¥SL. = ¥LV cos Q where YSV' YSL , and Ytv refer to the solid-vapour, solid-liquid, and liquid-vapour surface tensions res­ pectively, and Q is the contact angle formed by the liquid drop on the surface in a given atmosphere. This angle may be measured geometrically. Although experimental difficulties exist for the measurement of the various surface tensions, and stresses on the solid surface would confer non-equilibrium pro­ perties on the system, the measurement of Q is able to provide valuable information as to the relationships and affinities between the three phases. In particular, in the absence of chemical reactions at the interfaces, the shape of the liquid drop, and 6. hence the value of the contact angle, enables one to make an assessment of the "wettability" of the solid by the liquid. All solids are wet by all liquids to some extent, i.e.,• Q-<' 180;0 the greater the tendency of the liquid to spread on the solid, the smaller will Q become. When the liquid spreads completely over the solid, then Q equals zero. In the present study, attentionlas been directed to the wettability of baked amorphous carbon surfaces by liquid cryolite and its various alumina-containing solutions. It has been the intention to measure the extent of wettability as a function of carbon composi­ tion, particularly as affected by the addition 0f the inorganic additives related to the alumina reduction process. The work of Vajna (6) bas been followed up and used as a basis for further study, as described in de­ tail in the following sections. 7. (b) Experimental: (i) Anode Preparatisn ~Greenn or unbaked anodes were -- ' ' prepared on a laborat0ry scale by a method analagous to plant operati0n. Initially, this consisted of preparing a quantity Gf dry petroleum coke of the f0ll0wing particle size analysis:- t -1+ 4 mesh 17%- ), ) 4 + 20 mesh 33% ) - ) 20-+ 48 mesh 7"$ ) - ) Tyler Sieves 48 + 100--' mesh 8% ). - ) 1 00 -t:- 200: mesh 13% ) l 200 mesh 22% ). These percentages were selected from plant rec©rds over a twelve month period, and thus constituted a particle size distribution which had proved. satis­ factory in reducti0n furnace operation. A pre-determined quantity of the ab0ve coke mixture was heated t~ approximately 100°6 in a two-gallon double sig11a-bladed Baker-Perkins mixer, and 20% of its weight of coal-tar pitch, ©f softening point 105 - 110°c, added. Maintaining parity with plant conditions, the pitch-coke mixture was heated to 12~C, and a suitable quantity, mormally 150 - 160 grams transferred to a steel mould, 2" inside daameter and 3" long, lagged 8. with refractory paper, maintained at the same temperature. By means of a suitable plunger (See Fig.1), the hot mixture was compressed to a total pressure of 17,500 - 18,000 lb, or 5,570 - 5,730 lb per square inch, using a Mohr and Federhaff Universal Testing Machine.
Details
-
File Typepdf
-
Upload Time-
-
Content LanguagesEnglish
-
Upload UserAnonymous/Not logged-in
-
File Pages128 Page
-
File Size-