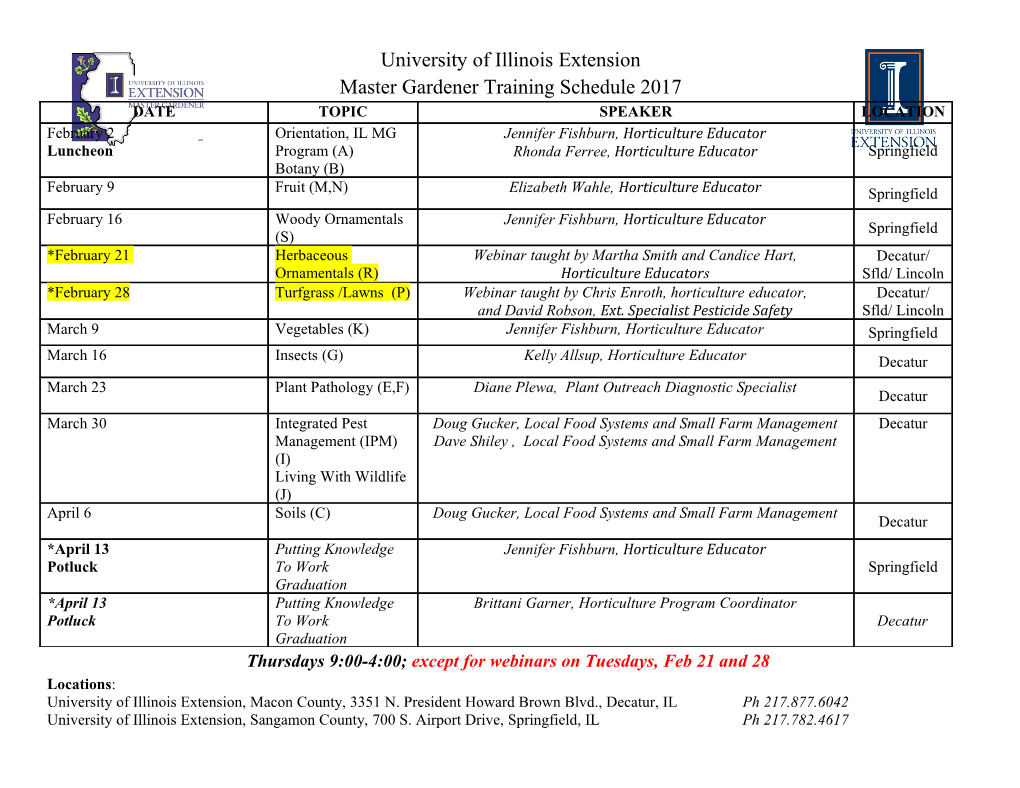
A&A 633, A41 (2020) Astronomy https://doi.org/10.1051/0004-6361/201936526 & c ESO 2020 Astrophysics Structure of a massive common envelope in the common-envelope wind model for Type Ia supernovae Ren Song1,2, Xiangcun Meng1,3,4 , Philipp Podsiadlowski5, and Yingzhen Cui1,2 1 National Astronomical Observatories/Yunnan Observatory, Chinese Academy of Sciences, Kunming 650216, PR China e-mail: [email protected], [email protected] 2 University of Chinese Academy of Sciences, Beijing 100049, PR China 3 Key Laboratory for the Structure and Evolution of Celestial Objects, Chinese Academy of Sciences, 650216 Kunming, PR China 4 Center for Astronomical Mega-Science, Chinese Academy of Sciences, 20A Datun Road, Chaoyang District, Beijing 100012, PR China 5 Department of Astronomy, Oxford University, Oxford OX1 3RH, UK Received 20 August 2019 / Accepted 13 November 2019 ABSTRACT Context. Although Type Ia supernovae (SNe Ia) are important in many astrophysical fields, the nature of their progenitors is still unclear. A new version of the single-degenerate model has been developed recently, the common-envelope wind (CEW) model, in which the binary is enshrouded in a common envelope (CE) during the main accretion phase. This model is still in development and has a number of open issues, for example what is the exact appearance of such a system during the CE phase? Aims. In this paper we investigate this question for a system with a massive CE. Methods. We use a thermally pulsing asymptotic giant branch (TPAGB) star with a CO core of 0.976 M and an envelope of 0.6 M to represent the binary system. The effects of the companion’s gravity and the rotation of the CE are mimicked by modifying the gravitational constant. The energy input from the friction between the binary and the CE is taken into account by an extra heating source. Results. For a thick envelope, the modified TPAGB star looks similar to a canonical TPAGB star but with a smaller radius, a higher effective temperature, and a higher surface luminosity. This is primarily caused by the effect of the companion’s gravity, which is the dominant factor in changing the envelope structure. The mixing length at the position of the companion can be larger than the local radius, implying a breakdown of mixing-length theory and suggesting the need for more turbulence in this region. The modified TPAGB star is more stable than the canonical TPAGB star and the CE density around the companion is significantly higher than that assumed in the original CEW model. Conclusions. Future work will require the modelling of systems with lower envelope masses and the inclusion of hydrodynamical effects during the CE phase. Key words. binaries: close – stars: evolution – supernovae: general – white dwarfs 1. Introduction involving the merging of two CO WDs (Tutukov & Yungelson 1981; Iben & Tutukov 1984; Webbink 1984). At present, both Type Ia supernovae (SNe Ia), some of the most violent explo- models have some theoretical and observational support. In this sions in the Universe, play an important role in a number of paper, we focus on a SD model. In the classical SD model, a astrophysical fields (Wang & Han 2012; Maoz et al. 2014). As CO WD accretes material from a non-degenerate companion star one of the best distance indicators, SNe Ia have been applied to increase its mass (Nomoto 1982). When the mass of the CO to measure cosmological parameters, which led to the discov- WD reaches a mass close to the Chandrasekhar limit, Mch, the ery of an accelerating expansion of the Universe (Riess et al. CO WD explodes as a SN Ia (Nomoto et al. 1984). In the SD 1998; Perlmutter et al. 1999). SNe Ia are also very important model, there is a critical accretion rate, M˙ cr. If the mass-transfer for the chemical evolution of galaxies as the main contributor of rate, M˙ tr, in the binary system exceeds M˙ cr, the WD expands iron neucleosynthesis (Matteucci & Greggio 1986). It is widely to form a RG-like object and then possibly swallows the com- believed that a SN Ia originates from the thermonuclear explo- panion to form a common envelope (CE, Nomoto 1982). The sion of a carbon-oxygen white dwarf (CO WD) in a binary sys- dynamics of the system during the CE phase are poorly under- tem (Hillebrandt et al. 2013), but the nature of the companion stood (Ivanova et al. 2013). The binary may lose its orbital angu- to the CO WD has remained controversial (Wang & Han 2012; lar momentum by friction with the CE and ultimately merge Maoz et al. 2014). rather than produce a SN Ia (Nomoto et al. 1979). On the other Depending on the companion’s nature, progenitor models of hand, if M˙ tr is too low, a nova explosion occurs and prevents the SNe Ia are mainly divided into two classes, the single-degenerate WD from gaining mass, again not leading to a SN Ia explosion (SD) model, in which the companion may be a main-sequence (Wang & Han 2012). Because of this fine-tuning, the birth rate (MS) star, a red giant (RG), or a helium star (Whelan & Iben of SNe Ia appears to be lower in the classical SD model than 1973;Nomoto et al.1984),andthedouble-degenerate(DD)model observed. Article published by EDP Sciences A41, page 1 of 10 A&A 633, A41 (2020) To increase the birth rate of SNe Ia in the SD model, Hachisu et al.(1996) developed the optically thick wind (OTW) model, which leads to a much higher SN Ia rate (Hachisu et al. 1999; Han & Podsiadlowski 2004), in fact comparable with the observed rate. In the OTW model, an OTW is assumed to occur when M˙ tr > M˙ cr, rather than the formation of a CE. The CO WD will then gradually increase its mass at the rate of M˙ cr and any unprocessed matter is blown off from the sur- face of the WD (Hachisu et al. 1996). However, some predic- tions from the OTW model are in conflict with observations. For example, because the OTW is driven by Fe lines, the OTW occurs only when Z ≥ 0:002, implying that there should be no SNe Ia in the early Universe or in low-metallicity environ- ments (Kobayashi et al. 1998). This cannot explain those SNe Ia that occur at high redshifts or in low-metallicity environments (Prieto et al. 2008; Rodney et al. 2015). In addition, the theoret- − ical OTW velocity (about 1000 km s 1, Hachisu et al. 1996) is Fig. 1. Schematic diagram illustrating the initial equivalent model dur- much higher than that inferred from observations of circumstel- ing the CE phase. The envelope is divided into three regions, the inner lar material (about 100 km s−1, Patat et al. 2007; Sternberg et al. co-rotating region (blue), the outer differentially rotating region (yel- 2011; Dilday et al. 2012). There are also some fine-tuning prob- low), and a transition region (light red) at the bottom of the outer region. lems in the OTW model, such as how to regulate simultane- The CO core represents the CO WD in the CEW model, in which stable hydrogen burning and helium shell flashes adjust themselves just as in ous (or alternating) helium and hydrogen burning (Piersanti et al. a normal TPAGB star. 2000; Shen & Bildsten 2007). To overcome the shortcomings of the OTW model, Meng & Podsiadlowski(2017; hereafter MP17) recently devel- 2. Numerical methods oped the common-envelope wind (CEW) model. In the CEW 2.1. Initial model model, when M˙ tr > M˙ cr a CE is assumed to form around the binary instead of an OTW. The CE is divided into two regions: In this study we use the Modules for Experiments in Stellar Astro- the inner region of the CE co-rotates with the binary, while the physics (MESA) code (version 10108; Paxton et al. 2011, 2013, outer region rotates differentially with the angular velocity drop- 2015, 2018) to carry out 1D hydrostatic simulations for systems ping off as a power law (following Meyer & Meyer-Hofmeister during the CE phase in the CEW model. As a first step in a series 1979). The CO WD increases its mass at the rate of M˙ cr. The of planned simulations, we simulate the structure of a binary sys- differentially rotating outer envelope extracts orbital angular tem with a massive CE as indicated by MP17. Because the system momentum from the inner binary into the outer region. Dur- is likely to look like a TPAGB star during the CE phase, we take a ing the CE phase, the system might look like a thermally puls- single TPAGB star as our starting model and simulate the effects of ing asymptotic giant branch (TPAGB) star in which alternating the spiraling-in companion in its envelope by changing the grav- phases of hydrogen and helium burning self-adjust just as they itational potential in the TPAGB star to imitate the effect of the do in TPAGB stars (MP17). The CEW model may resolve some companion’s gravity. This is a simple approximation, which we of the problems of the OTW model but also retain many of its discuss further in Sect.4. At present, three e ffects are considered: merits, such as only a weak dependence on the metallicity, and (1) the energy input from the secondary and the friction between the wind velocity from the CE surface is expected to be less than the binary system and the CE, (2) the gravity from the companion, about 200 km s−1, as inferred from observations.
Details
-
File Typepdf
-
Upload Time-
-
Content LanguagesEnglish
-
Upload UserAnonymous/Not logged-in
-
File Pages10 Page
-
File Size-