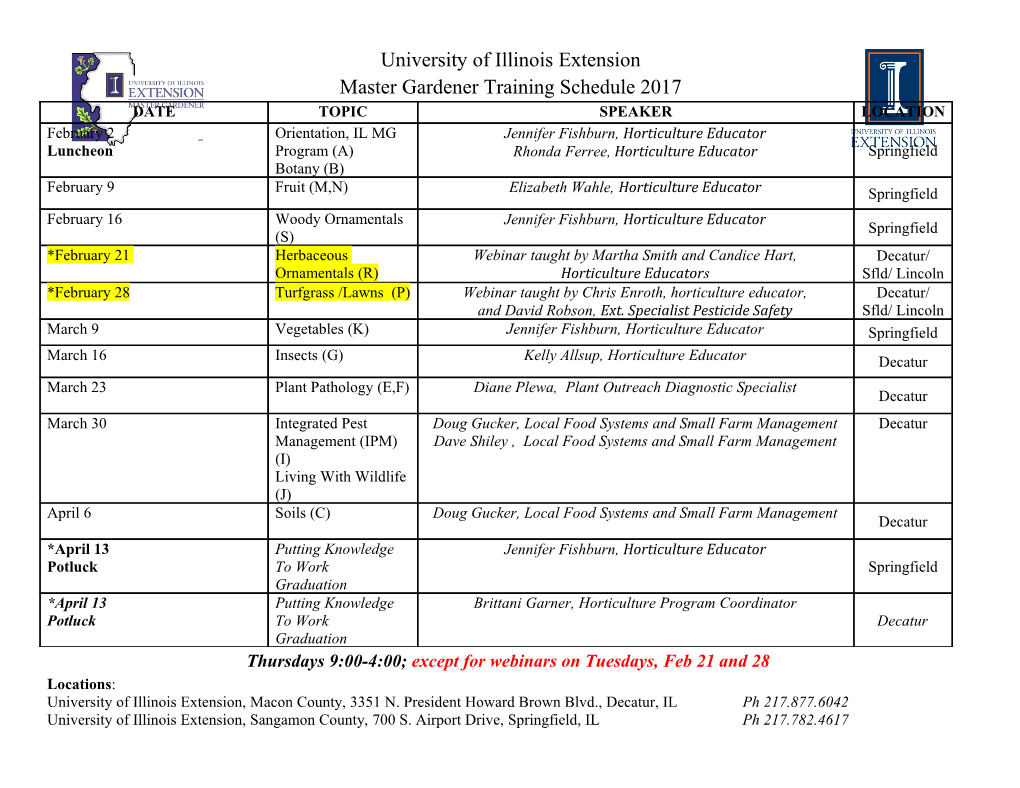
UvA-DARE (Digital Academic Repository) Group 9 open-shell organometallics: reactivity at the ligand Dzik, W.I. Publication date 2011 Document Version Final published version Link to publication Citation for published version (APA): Dzik, W. I. (2011). Group 9 open-shell organometallics: reactivity at the ligand. General rights It is not permitted to download or to forward/distribute the text or part of it without the consent of the author(s) and/or copyright holder(s), other than for strictly personal, individual use, unless the work is under an open content license (like Creative Commons). Disclaimer/Complaints regulations If you believe that digital publication of certain material infringes any of your rights or (privacy) interests, please let the Library know, stating your reasons. In case of a legitimate complaint, the Library will make the material inaccessible and/or remove it from the website. Please Ask the Library: https://uba.uva.nl/en/contact, or a letter to: Library of the University of Amsterdam, Secretariat, Singel 425, 1012 WP Amsterdam, The Netherlands. You will be contacted as soon as possible. UvA-DARE is a service provided by the library of the University of Amsterdam (https://dare.uva.nl) Download date:04 Oct 2021 Group 9 Open-Shell Organometallics: Reactivity at the Ligand Wojciech I. Dzik 2011 Group 9 Open-Shell Organometallics: Reactivity at the Ligand Wojciech Invitation Group 9 to the public defence of my Thesis Open-Shell Organometallics: which will take place on: Reactivity at the Ligand Tuesday, 11th of January 2011 at 10:00 in the Agnietenkapel Oudezijds Voorburgwal 231 Amsterdam After the ceremony a reception will take place Wojciech Dzik [email protected] Paranymphs: Erica Jellema [email protected] Paweł Dydio Wojciech I. Dzik [email protected] Group 9 Open-Shell Organometallics: Reactivity at the Ligand Wojciech I. Dzik Cover: Radical reaction (taking place on a redox non-innocent ligand) approaching the transition state. Photo: © Adam Gryko / istockphoto.com Group 9 Open-Shell Organometallics: Reactivity at the Ligand ACADEMISCH PROEFSCHRIFT ter verkrijging van de graad van doctor aan de Universiteit van Amsterdam op gezag van de Rector Magnificus prof. dr. D.C. van den Boom ten overstaan van een door het college voor promoties ingestelde commissie, in het openbaar te verdedigen in de Agnietenkapel op dinsdag 11 januari 2011, te 10:00 uur door Wojciech Iwo Dzik geboren te Columbus, Verenigde Staten van Amerika Promotiecommissie Promotor: Prof. dr. J. N. H. Reek Co-promotor: Dr. B. de Bruin Overige Leden: Prof. dr. C. J. Elsevier Dr. D. G. H. Hetterscheid Prof. dr. K. Lammertsma Dr. ir. J. I. van der Vlugt Prof. dr. K. Wieghardt Prof. dr. X. P. Zhang Faculteit der Natuurwetenschappen, Wiskunde en Informatica The research described in this dissertation has been financially supported by the Netherlands Organization for Scientific Research – Division Chemical Sciences (NWO- CW) within VIDI project 700.55.426 Table of Contents Chapter 1 General Introduction: Redox Non-Innocent Behavior of 1 Alkene, Carbonyl and Carbene Ligands Chapter 2 Activation of Carbon Monoxide by (Me3tpa)Rh and 21 (Me3tpa)Ir Chapter 3 Carbonyl Complexes of Rhodium with N-donor Ligands: 49 Factors Determining the Formation of Terminal versus Bridging Carbonyls Chapter 4 Base Assisted Selective Reduction of Organometallic 69 RhII and IrII Radicals Chapter 5 Selective C–C Coupling of Ir-Ethene and Ir-Carbene 87 Radicals Chapter 6 ‘Carbene Radicals’ in CoII(por)-Catalyzed Olefin 99 Cyclopropanation Chapter 7 Hydrogen Atom Transfer in Reactions of Organic 121 Radicals with (por)CoII• and in Subsequent Addition of (por)Co–H to Olefins Chapter 8 Perspective: Ligand Radicals in New, Catalytic Reactions 137 Summary 143 Samenvatting 149 Streszczenie 155 List of Publications 161 Acknowledgements 163 Chapter 1 General Introduction: Redox Non-Innocent Behavior of Alkene, Carbonyl and Carbene Ligands Chapter 1 1.1 Introduction Radicals are often considered to be too reactive to be selective. For free radicals this might be true, but in the coordination sphere of a transition metal highly chemo-, regio-, diastereo- and enantioselective radical-type reactions become feasible. Selectivity is primarily a matter of relative rather than absolute rates, and hence also fast radical-type reactions can be (made) selective using the steric and electronic influence of ligand surroundings in transition metal controlled reactions.1 Nature is teaching us clearly that selective radical reactions are certainly possible and radical-type reactions are actually quite common in several biochemical processes. Many metallo-enzyme catalyzed transformations proceed via radical-type reactions involving open-shell intermediates. They are essential in mediating difficult reactions such as C–C or C–O bond formations or transformations, and despite their radical nature these reactions still proceed in the highly selective manner typical for enzymatic reactions.2 Selective radical-type atom transfer reactions most frequently require the presence of unpaired electron (radical) density at a reactive ligand site (e.g. oxo / oxyl radical ligands),3 as is clear from mechanistic studies of bio-catalytic processes mediated 4 5 by among others cytochrome P450, methane monooxygenases, class I ribonucleotide reductase6 or galactose oxidase. Tyr S S Tyr OH O O His O N II N II Cu H Cu H N His N O R O R H H H H2O2 RCH2OH S S OH OH HO N I N II O Cu Cu O O2 N O N O O H H H R R Figure 1. Cooperative ligand radical in the mechanism of alcohol oxidation by galactose oxidase. The mechanism of Galactose Oxidase (GOase) is perhaps one of the most illustrative examples in showing how radicals can be controlled in the coordination sphere of a transition metal to achieve selectivity (Figure 1).7 This fungal enzyme chemo-selectively converts primary alcohols with O2 into aldehydes and hydrogen peroxide with impressive second order rate constants (~1.59 x 104 M−1 s−1).8 The active site of GOase consists of a redox active CuI ion in proximity to a redox active cysteine modified tyrosine. Oxidation of the active site by dioxygen produces a 2 General Introduction tyrosinyl radical coordinated to CuII and a hydroperoxo ligand. In the key subsequent steps of the catalytic cycle the thus obtained hydroperoxo ligand deprotonates the alcohol to form free hydrogen peroxide and an alcoholate ligand. The tyrosinyl radical ligand subsequently abstracts a hydrogen atom from the α-CH functionality of the alcoholate to produce the aldehyde. Remarkably, the tyrosinyl radical is a reactive fragment in the catalytic cycle and functions as a cooperative ligand, assisting copper in the turn-over process. The ligand redox activity allows removal of the second electron from the substrate, and thereby a two-electron oxidation using a copper ion that is normally only capable of one-electron transformations. It is the interplay of the one-electron reactivity of the ligand radical and the one-electron reactivity of the metal which makes the overall two-electron oxidation process possible. The cooperative radical-type process makes the reaction fast and selective. Metal-ligand bond homolysis within an enzyme pocket is another frequently observed pathway to start selective radical-type transformations. The typical radical-type 9 reactions mediated by cobalamine (coenzyme B12) based dependent mutases (allowing carbon skeleton rearrangements)10 and diol dehydrase or ethanolamine deaminase11 are initiated by homolytic splitting of a weak cobalt(III)-carbon bond (Figure 2).12 The thus formed carbon radical serves as a hydrogen atom acceptor from the substrate CH group. After the first hydrogen atom transfer (HAT) step the open shell substrate rearranges through the migration of a functional group to the radical carbon followed by the second HAT to form the closed-shell rearranged product. The •CH2Ad radical remains in close proximity to the cobalt(II) centre reforming the cobalt-carbon bond after the catalytic turnover. H2NOC C C CONH2 H X CH2Ad CH2Ad H2NOC III II N R N CONH2 Co Co Co H N N N N H2NOC CONH2 C C X H O N NH N C C C C HO O X X O P CH Ad CH Ad O O 3 3 O OH CoII CoII N O NH N N H2C N 2 R=CH2Ad = N N HO OH Figure 2. Structure of coenzyme B12 and the generic mechanism for AdoCbl-dependent rearrangement reactions. 3 Chapter 1 The mechanisms of reactions catalyzed by galactose oxidase or coenzyme B12 are excellent examples of how radical-type reactions can be controlled in the coordination sphere of a transition metal, and serve as a clear inspiration for synthetic chemists. Interesting bio-mimetic approaches to GOase have already shown synthetic power in alcohol oxidation reactions,13 but future studies are likely to uncover a wide field of new opportunities. The ease of reversible generation of a carbon centered radical by the cleavage of the weak Co–C bond (used by Nature in the coenzyme B12) has found wide application in transition metal controlled radical polymerization.14 The latter example shows elegantly that the same phenomena (i.e. homolytic cleavage of a weak metal- carbon bond) that are used in enzymes for certain transformation in vivo can be successfully applied to mediate reactions (like polymerization of olefins) that occur only in chemical laboratories. Some other important examples of synthetic (catalytic) reactions that proceed via open-shell intermediates are atom transfer radical polymerization,14a atom transfer radical addition,15 and oxygenation reactions.16 For most of these reactions, atom or group transfer to a reactive metal-centered radical (metallo-radical) occurs at some stage of the catalytic reaction, but properly designed synthetic transition metal complexes should allow us to activate substrates in a similar (cooperative) way as Nature does.
Details
-
File Typepdf
-
Upload Time-
-
Content LanguagesEnglish
-
Upload UserAnonymous/Not logged-in
-
File Pages173 Page
-
File Size-