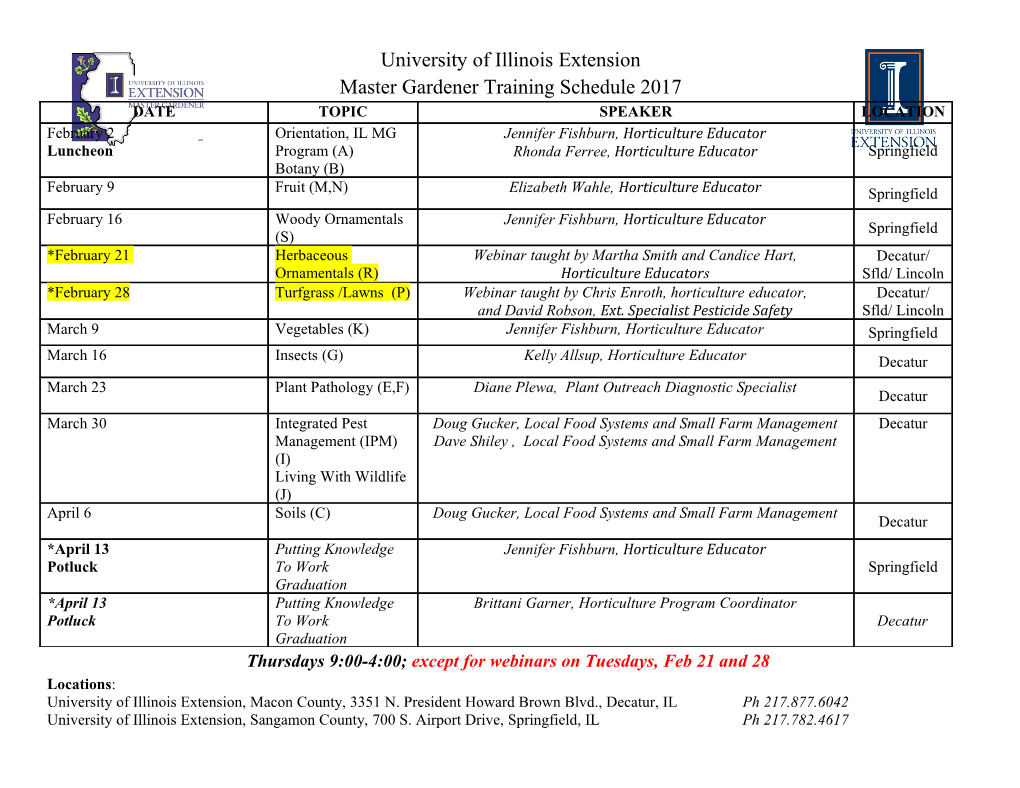
Rheological change and degassing during a trachytic Vulcanian eruption at Kilian Volcano, Chaîne des Puys, France Mathieu Colombier, Thomas Shea, Alain Burgisser, Timothy Druitt, Lucia Gurioli, Dirk Müller, Francisco Cáceres, Kai-Uwe Hess, Pierre Boivin, Didier Miallier, et al. To cite this version: Mathieu Colombier, Thomas Shea, Alain Burgisser, Timothy Druitt, Lucia Gurioli, et al.. Rheological change and degassing during a trachytic Vulcanian eruption at Kilian Volcano, Chaîne des Puys, France. Bulletin of Volcanology, Springer Verlag, 2020, 82 (12), 10.1007/s00445-020-01420-5. hal- 03015892v1 HAL Id: hal-03015892 https://hal.archives-ouvertes.fr/hal-03015892v1 Submitted on 20 Nov 2020 (v1), last revised 25 Nov 2020 (v2) HAL is a multi-disciplinary open access L’archive ouverte pluridisciplinaire HAL, est archive for the deposit and dissemination of sci- destinée au dépôt et à la diffusion de documents entific research documents, whether they are pub- scientifiques de niveau recherche, publiés ou non, lished or not. The documents may come from émanant des établissements d’enseignement et de teaching and research institutions in France or recherche français ou étrangers, des laboratoires abroad, or from public or private research centers. publics ou privés. Manuscript Click here to access/download;Manuscript;Final Kilian II- resubmission.docx Click here to view linked References 1 Rheological change and degassing during a trachytic Vulcanian 2 eruption at Kilian Volcano, Chaîne des Puys, France 3 Mathieu Colombier1; Thomas Shea2; Alain Burgisser3; Timothy H. Druitt4; Lucia Gurioli4; Dirk 4 Müller1; Francisco Cáceres1; Kai-Uwe Hess1; Pierre Boivin4; Didier Miallier5; and Donald B. 5 Dingwell1 6 1Department of Earth and Environmental Sciences, Ludwig-Maximilians-Universität 7 München, Germany; 2Geology and Geophysics, University of Hawai'i at Mānoa, Honolulu, 8 Hawaii, USA; 3Univ. Savoie Mont Blanc, CNRS, IRD, ISTerre, F-73376 Le Bourget du Lac, 9 France; 4Université Clermont Auvergne -CNRS-IRD, OPGC, Laboratoire Magmas et Volcans, 10 F-63100, Clermont-Ferrand, France; 5 Université Clermont Auvergne, CNRS–IN2P3, LPC, F- 11 63000 Clermont-Ferrand, France 12 13 Keywords: Effusive-explosive transitions, Trachytic magma, Vulcanian eruption, Viscosity, 14 Crystallization, Degassing, Nanolites, Cristobalite. 15 16 Abstract 17 Magma ascent during silicic dome-forming eruptions is characterized by significant changes in 18 magma viscosity, permeability and gas overpressure in the conduit. These changes depend on 19 a set of parameters such as ascent rate, outgassing and crystallization efficiency, and magma 20 viscosity which in turn may influence the prevailing conditions for effusive versus explosive 21 activity. Here, we combine chemical and textural analyses of tephra with viscosity models to 22 provide a better understanding of the effusive-explosive transitions during Vulcanian phases of 23 the 9.4 ka eruption of Kilian Volcano, Chaîne des Puys, France. Our results suggest that effusive 24 activity at the onset of Vulcanian episodes at Kilian Volcano was promoted by (i) rapid ascent 25 of initially crystal-poor and volatile-rich trachytic magma, (ii) a substantial bulk and melt 26 viscosity increase driven by extensive volatile loss and crystallization, and (iii) efficient 27 degassing/outgassing in a crystal-rich magma at shallow depths. Trachytic magma repeatedly 28 replenished the upper conduit, and variations in the amount of decompression and cooling 29 caused vertical textural stratification, leading to variable degrees of crystallization and 30 outgassing. Outgassing promoted effusive dome growth and occurred via gas percolation 31 through large interconnected vesicles, fractures and tuffisite veins, fostering the formation of 32 cristobalite in the carapace and talus regions. Build-up of overpressure was likely caused by 33 closing of pore space (bubbles and fractures) in the dome through a combination of pore 34 collapse, cristobalite formation, sintering in tuffisite veins or limited pre-fragmentation 35 coalescence in the dome or underlying hot vesicular magma. Sealing of the carapace may have 36 caused a transition from open to closed system degassing and to renewed explosive activity. 37 We generalize our findings to propose that the broad spectrum of eruptive styles for trachytic 38 magmas may be inherited from a combination of characteristics of trachytic melts, that include 39 high water solubility and diffusivity, rapid microlite growth, and low melt viscosity compared 40 to their more evolved subalkaline dacitic and rhyolitic equivalents. We show that trachytes may 41 erupt with a similar style (e.g., Vulcanian) but at significantly higher ascent rates than their 42 andesitic, dacitic and rhyolitic counterparts. This suggests that the periodicity of effusive- 43 explosive transitions at trachytic volcanoes may differ from that observed at the well-monitored 44 andesitic, dacitic and rhyolitic volcanoes, which has implications for hazard assessment 45 associated with trachytic eruptions. 46 47 Introduction 48 Understanding and forecasting effusive-explosive transitions during intermediate-to-silicic 49 volcanic eruptions is a key objective of volcanology. Such transitions occur frequently (e.g., 50 Cassidy et al. 2018), yet they remain difficult to anticipate. During andesitic, dacitic and 51 trachytic eruptions, effusive phases commonly lead to the emplacement of crystal-rich lava 52 domes or plugs stalling at shallow levels of the conduit (Platz et al. 2007; Lavallée et al. 2012; 53 Kushnir et al. 2016; Colombier et al. 2017a; Heap et al. 2018). Explosive activity and conduit 54 evacuation can in turn be triggered by the pressurization of underlying vesicular magma which 55 ultimately yields a downward propagation of a fragmentation front within the conduit (e.g., 56 Druitt et al. 2002; Platz et al. 2007; Giachetti et al. 2010; Calder et al. 2015) or by over- 57 pressurization of the dome itself (e.g., Boudon et al. 2015). As a result of these transitions, 58 dense, crystal-rich, dome-derived pyroclasts and highly vesicular, conduit-derived pumice 59 pyroclasts commonly coexist in the deposits of Vulcanian eruptions leading to bimodal 60 porosity/density distributions of the pyroclasts (Formenti and Druitt 2003; Adams et al. 2006; 61 Mujin and Nakamura 2014; Colombier et al. 2017a). Crystallization during magma ascent, and 62 stagnation at shallow levels, is mostly driven by processes related to decompression, degassing 63 and/or cooling (e.g., Blundy and Cashman 2001; Arzilli and Caroll 2013; Zorn et al. 2018). 64 Effusive-explosive transitions have been proposed to arise from differences in magma 65 ascent rate (e.g., Platz et al. 2007; Cassidy et al. 2018), degassing conditions (e.g., Eichelberger 66 et al. 1986; Jaupart and Allègre 1991), pre- or syn-eruptive volatile content (e.g., Andujar and 67 Scaillet 2012; Forte and Castro 2019), rheological changes in the magma (e.g., Moitra et al. 68 2018; Cassidy et al. 2018) and lava-water interaction (e.g., Belousov et al. 2011; Fitch et al. 69 2017). The emplacement of lava domes is typically associated with significant gas loss from 70 the magma by outgassing through connected bubble networks, fractures or intergranular pore 71 space (Kendrick et al. 2016; Colombier et al. 2017b; Lamur et al. 2017; Yoshimura et al. 2019). 72 Magma permeability is the dominant factor controlling the ability of gas to escape from the 73 magma, and its evolution is both complex and highly transient in lava domes (e.g., Mueller et 74 al. 2005). Rheological changes in the lava dome also have an important role in controlling cyclic 75 and hybrid eruptive style (e.g., Lavallée et al. 2013). They are principally governed by the 76 kinetics of crystallization, bubble nucleation and growth, and variations in volatile content and 77 temperature (cooling or heating). All these processes may have competing effects on eruptive 78 style. As an example, an increase in magma viscosity may (1) lead to a slower ascent rate, which 79 in turn may promote gas escape, magma cooling and effusive activity, or (2) have the adverse 80 effect of causing gas overpressure and magma brittle fragmentation, leading to explosive 81 eruption. Finally, ascent rate controls the time available for the formation of a permeable 82 magma. Faster ascent rates offer less time for permeable gas pathways to develop, thereby 83 favouring explosive activity (Cassidy et al. 2018). 84 Trachytic (and phonolitic) silicate melts differ strongly from their more widespread, 85 subalkaline counterparts (andesites, dacites and rhyolites) in several ways. Firstly, they have 86 lower viscosities at similar conditions of SiO2 content, temperatures and dissolved H2O than 87 rhyolitic melts due to differences in melt compositions (e.g., Andujar and Scaillet 2012). 88 Secondly, trachytic melts are inferred to crystallize faster than subalkaline compositions, with 89 higher crystal nucleation and growth rates leading to higher crystallinities and crystal number 90 densities on a given timescale (Arzilli and Caroll 2013; Shea et al. 2017). As crystals are known 91 to promote permeable gas escape at lower porosities (e.g., Blower 2001; Oppenheimer et al. 92 2015; Colombier et al. 2017b; Lindoo et al. 2017; Colombier et al. 2020), one might expect that 93 the timing and efficiency of outgassing are strongly influenced by the high rates of 94 crystallization experienced by trachytic magmas during ascent. Such rapid crystallization
Details
-
File Typepdf
-
Upload Time-
-
Content LanguagesEnglish
-
Upload UserAnonymous/Not logged-in
-
File Pages83 Page
-
File Size-