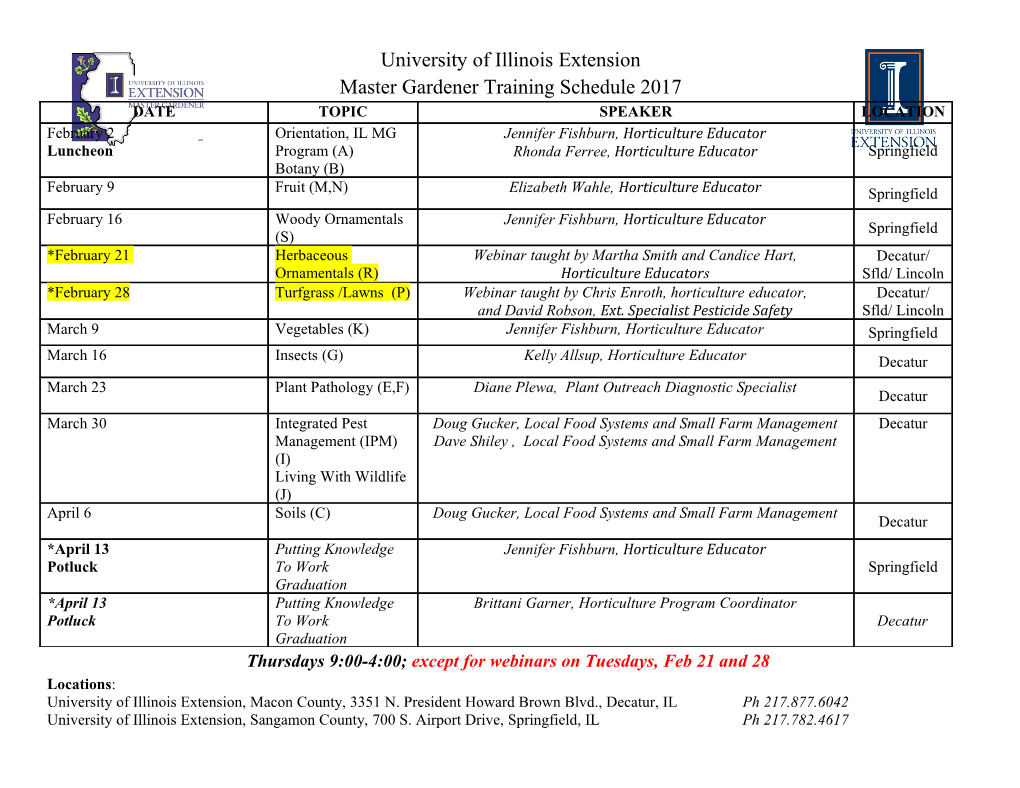
2784 Langmuir 2007, 23, 2784-2791 Self-Assembly and Phase Behavior of Germanium Oxide Nanoparticles in Basic Aqueous Solutions Jeffrey D. Rimer, Daniel D. Roth, Dionisios G. Vlachos,* and Raul F. Lobo* Center for Catalytic Science and Technology, Department of Chemical Engineering, UniVersity of Delaware, Newark, Delaware 19716 ReceiVed September 26, 2006. In Final Form: NoVember 20, 2006 We show that germania nanoparticle self-assembly in basic aqueous solutions occurs at a critical aggregation - concentration (CAC) corresponding to a 1:1 GeO2/OH molar ratio. A combination of pH, conductivity, and small- angle X-ray scattering (SAXS) measurements was used to monitor the effect of incremental additions of germanium (IV) ethoxide to basic solutions of sodium hydroxide or tetraalkylammonium cations. Plots of pH versus total germania concentration at varying alkalinities generated a phase diagram with three distinct regions. The diagram was analyzed with a thermodynamic model based on the chemical equilibria of germania speciation and dissociation. The model, which uses the GeO-H dissociation constant (pK ) 7.1) as the single fitting parameter, quantitatively captures trends in the CAC and pH. SAXS patterns reveal that the germania nanoparticles have either a cubic or a spherical geometry of dimension ∼1 nm that is independent of solution pH and cation. On the basis of these and other literature findings, we propose that the germania nanoparticle structure is that of the cubic octamer (double four-membered ring, Ge8O12- (OH)8), which is common among condensed GeO2 materials and building units in [Ge,Si]-zeolites. Comparisons between germania and silica solutions show distinct differences in their phase behavior and nanoparticle structure. The results presented here, in combination with previous studies of siliceous solutions, provide a framework for ongoing studies of combined germania-silica phase behavior, which is part of an overarching effort to understand the influence of heteroatoms in the growth and structure direction of zeolites. 1. Introduction AST;11 BEC and LTA;12 UOZ;13 EUO;14 RHO, NAT, GIS, and 15 16,17 18-20 The unique properties of germanium have led to the develop- FAU; BEA; and the UCSB series. A significant amount ment of Ge-containing zeolites, with over 20 framework types of effort has been focused on incorporating Ge into the ITQ series.2,14,21-28 The presence of Ge causes an expansion of the reported in the literature. The incorporation of Ge in the zeolite 6 framework drives the formation of large (12-membered rings, unit cell, without significantly affecting the zeolite acidity. Many MR) and extra-large (>12-MR) pores or channels. Subsequently, studies have focused on the Ge/Si ratio, where it is found that these materials could find extensive use in petroleum refining, in some zeolites (e.g., MFI framework), isomorphic substitution ion exchange, and separations, due to the increased accessibility by larger molecules. In particular, microporous materials with (8) Kosslick, H.; Tuan, V. A.; Fricke, R.; Peuker, C.; Pilz, W.; Storek, W. J. Phys. Chem. 1993, 97, 5678. open framework structures have received much attention for (9) Li, S.; Tuan, V. A.; Falconer, J. L.; Noble, R. D. Micropor. Mesopor. their widespread potential in separations of large molecules, such Mater. 2003, 58, 137. as heavy oils and pharmaceuticals.1 Corma and co-workers (10) Tuan, V. A.; Falconer, J. L.; Noble, R. D. Micropor. Mesopor. Mater. 2000, 41, 269. synthesized a series of [Si,Ge]-zeolites with an ISV framework (11) O’Keeffe, M.; Yaghi, O. M. Chem.sEur. J. 1999, 5, 2796. type consisting of large channels, with unique properties for (12) Sastre, G.; Pulido, A.; Corma, A. Micropor. Mesopor. Mater. 2005, 82, 2 159. applications in oil refinery and petrochemical processes. In (13) Mathieu, Y.; Paillaud, J. L.; Caullet, P.; Bats, N. Micropor. Mesopor. addition, Ge-zeolites have shown potential for enhancing catalytic Mater. 2004, 75, 13. activity, particularly in ZSM-5 catalysts where Ge incorporation (14) Sastre, G.; Pulido, A.; Castaneda, R.; Corma, A. J. Phys. Chem. B 2004, 108, 8830. reduces deactivation by introducing a higher mesoporosity and (15) Johnson, G. M.; Tripathi, A.; Parise, J. B. Chem. Mater. 1999, 11, 10. leads to a greater number of defects (i.e., hydroxyl groups) without (16) Corma, A.; Navarro, M. T.; Rey, F.; Rius, J.; Valencia, S. Angew. Chem., altering the overall topology.3 Intl. Ed. 2001, 40, 2277. (17) Corma, A.; Navarro, M. T.; Rey, F.; Valencia, S. Chem. Commun. 2001, Germanium has been incorporated into both mesoporous 1486. materials4,5 as well as numerous zeolitic frameworks: MFI;3,6-10 (18) Bu, X. H.; Feng, P. Y.; Stucky, G. D. J. Am. Chem. Soc. 1998, 120, 11204. (19) Bu, X. H.; Feng, P. Y.; Stucky, G. D. Chem. Mater. 2000, 12, 1505. (20) Gier, T. E.; Bu, X. H.; Feng, P. Y.; Stucky, G. D. Nature 1998, 395, 154. * Corresponding authors. (R.F.L.) Tel.: (302) 831-1261; e-mail: (21) Corma, A.; Diaz-Cabanas, M. J.; Garcia, H.; Palomares, E. Chem. Commun. [email protected].(D.G.V.)Tel.: (302)831-2830;e-mail: [email protected]. 2001, 2148. (1) Zhou, Y. M.; Zhu, H. G.; Chen, Z. X.; Chen, M. Q.; Xu, Y.; Zhang, (22) Blasco, T.; Corma, A.; Diaz-Cabanas, M. J.; Rey, F.; Vidal-Moya, J. A.; H. Y.; Zhao, D. Y. Angew. Chem., Intl. Ed. 2001, 40, 2166. Zicovich-Wilson, C. M. J. Phys. Chem. B 2002, 106, 2634. (2) Corma, A.; Diaz-Cabanas, M. J.; Fornes, V. Angew. Chem., Intl. Ed. 2000, (23) Corma, A.; Puche, M.; Rey, F.; Sankar, G.; Teat, S. J. Angew. Chem., 39, 2346. Intl. Ed. 2003, 42, 1156. (3) van de Water, L. G. A.; van der Waal, J. C.; Jansen, J. C.; Maschmeyer, (24) Corma, A.; Diaz-Cabanas, M. J.; Rey, F.; Nicolooulas, S.; Boulahya, K. T. J. Catal. 2004, 223, 170. Chem. Commun. 2004, 1356. (4) Lu, Q. Y.; Gao, F.; Li, Y. Q.; Zhou, Y. M.; Zhao, D. Y. Micropor. Mesopor. (25) Alvaro, M.; Atienzar, P.; Corma, A.; Ferrer, B.; Garcia, H.; Navarro, Mater. 2002, 56, 219. M. T. J. Phys. Chem. B 2005, 109, 3696. (5) Zou, X. D.; Conradsson, T.; Klingstedt, M.; Dadachov, M. S.; O’Keeffe, (26) Blasco, T.; Corma, A.; Diaz-Cabanas, M. J.; Rey, F.; Rius, J.; Sastre, G.; M. Nature 2005, 437, 716. Vidal-Moya, J. A. J. Am. Chem. Soc. 2004, 126, 13414. (6) van de Water, L. G. A.; van der Waal, J. C.; Jansen, J. C.; Cadoni, M.; (27) Castaneda, R.; Corma, A.; Fornes, V.; Rey, F.; Rius, J. J. Am. Chem. Soc. Marchese, L.; Maschmeyer, T. J. Phys. Chem. B 2003, 107, 10423. 2003, 125, 7820. (7) van de Water, L. G. A.; Zwijnenburg, M. A.; Sloof, W. G.; van der Waal, (28) Corma, A.; Rey, F.; Rius, J.; Sabater, M. J.; Valencia, S. Nature 2004, J. C.; Jansen, J. C.; Maschmeyer, T. Chem. Phys. Chem. 2004, 5, 1328. 431, 287. 10.1021/la062828q CCC: $37.00 © 2007 American Chemical Society Published on Web 01/23/2007 Self-Assembly and Phase BehaVior of Germanium Oxide Langmuir, Vol. 23, No. 5, 2007 2785 monium (TMA+)sa molecule that has also been identified as being a unique component in the self-assembly of silica into cubic octamers.39-42 Much of the work done on Ge-zeolites has been focused on structural characterization, but the effects of germanium on the kinetics and mechanism of zeolite growth are not well-understood. There lacks a systematic study of the aqueous chemistry of germania and germania-silica solutions, which is needed to develop insight into the effects of Ge incorporation for the rational design of new materials. In this paper, we focus on all-germania aqueous solutions and present an analysis of the phase behavior through a combination of pH, conductivity, and small-angle X-ray scattering (SAXS) measurements. A chemical equilibrium model, based on germania dissociation and condensation, is used to predict both the phase diagram and the onset of nanoparticle self-assembly. The results of these analyses serve as a basis for Figure 1. Zeolitic frameworks with double four-membered ring 23 29 30,31 11 studying mixed Ge/Si phase behavior, thereby providing a subunits for ITH, UTL, LTA, and AST. foundation for future mechanistic studies of [Ge,Si]-zeolite crystallization. of germanium can replace more than 30% of the silicon atoms, with an upper limit of 12.8 per unit cell.8 In materials such as 2. Experimental Procedures ITQ-7, it has been suggested by Blasco et al. that a maximum Germania nanoparticles in basic solutions of monovalent cations of two or three Ge atoms can occupy a double four-membered were synthesized by first diluting concentrated tetramethylammonium ring (D4R).22 hydroxide (TMAOH, 25% w/w, Alfa Aesar), tetrapropylammonium hydroxide (TPAOH, 40% w/w, Alfa Aesar), or sodium hydroxide The probability of forming frameworks with D4R subunits is 23,27,28,32-34 in deionized water. Solutions of the latter were obtained by diluting greatly enhanced by the presence of Ge, due to the a 5.0 M stock solution of sodium hydroxide (NaOH, Aldrich, pellets, - - increase in bond flexibility, since the optimum Ge O Ge bond 97+ %, ACS) to attain the desired alkalinity. After mixing for ∼30 angle of 130° is smaller than the 145° angle of the Si-O-Si min, germanium (IV) ethoxide (99.95+%, Aldrich) was added to bond,11,25 as well as the longer Ge-O distance.35 Figure 1 shows each solution, and the resulting mixture was vigorously stirred for examples of various Ge-zeolite frameworks comprised of D4R at least 12 h prior to analysis.
Details
-
File Typepdf
-
Upload Time-
-
Content LanguagesEnglish
-
Upload UserAnonymous/Not logged-in
-
File Pages8 Page
-
File Size-