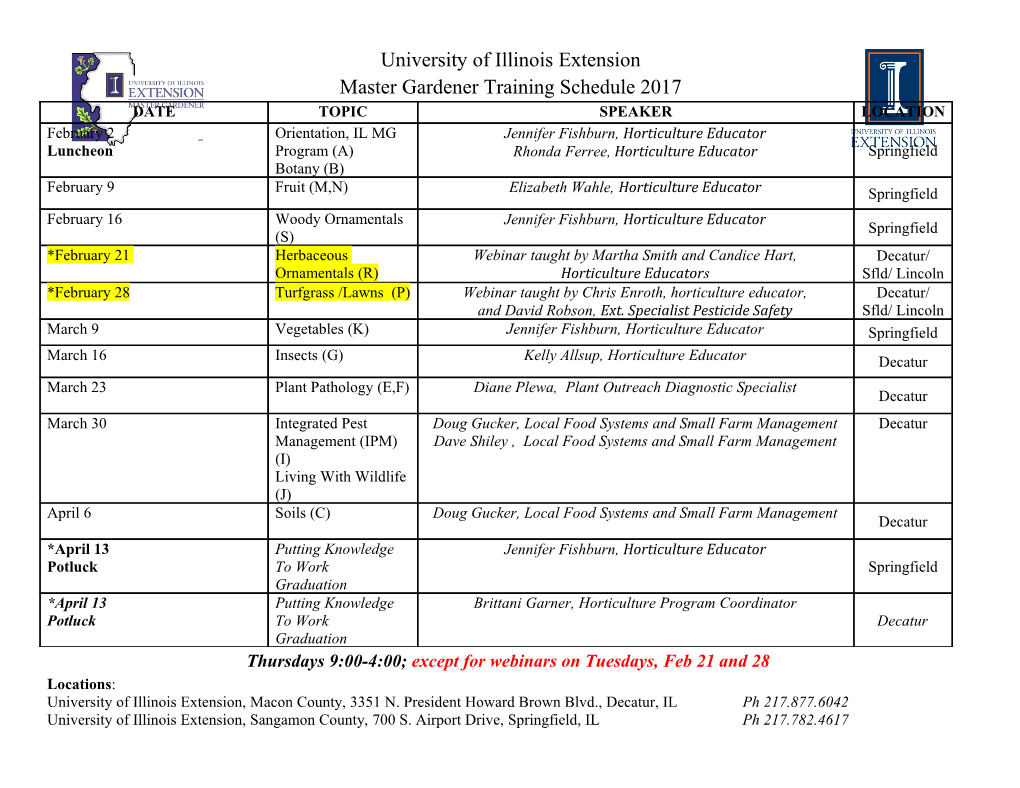
10 th International Conference on Hydrodynamics October 1-4, 2012 St. Petersburg, Russia Propulsion and Steering Behaviour of a Ship Equipped with Two Contra-Rotating Z-drives Manases Tello Ruiz 1, Guillaume Delefortrie *2 , Marc Vantorre 1, Stefan Geerts 1 1Ghent University 2Flanders Hydraulics Research Ghent, Belgium Antwerp, Belgium * E-mail: [email protected] Such systems have mostly been implemented for ABSTRACT . dynamic DP systems and as a main propulsive unit for tugs which are constrained to operability ranges of In 2011 captive manoeuvring tests have been carried out with a 1/25 scale model of an estuary vessel in the towing tank for low thruster advance numbers and flat bottom hull manoeuvres in shallow water (co-operation Flanders form shape. Different methods addressed to solve the Hydraulics Research – Ghent University). As this vessel was hydrodynamic forces of azimuth drives can be found equipped with two contra-rotating Z-drives, a significant part of in the literature, based on experimental and theoretical the testing program focussed on the propulsion and steering researches [2-6], as well as on numerical methods [7]. behaviour of this system, by repeating tests at different azimuth angles and propeller rates. These tests enabled the assessment of the interaction behaviour between the two drives and their Nienhuis [2] and Cozijn et al. [8] analysed the thruster effect on the propulsion and steering behaviour of the ship. In effectiveness for azimuth drives for open water cases this way a mathematical model could be developed for and mounted behind a flat barge. These scenarios implementation in a full mission inland ship manoeuvring were also studied by Stettler [4] with a ship hull form simulator. The paper intends to give a description of the test- and a single propeller but no ship-thruster interaction setup followed by an extensive discussion of the effect the Z- was studied since the units were designed in a way the drives on the manoeuvring behaviour of the estuary vessel. hull wake and boundary layer are outside the propeller slipstream. KEY WORDS : Contra-rotating Z-drives, azimuth thruster, thruster-thruster interaction, ship-thruster interaction. The installation of azimuth drives in an estuary vessel requires more concern about the overall operability INTRODUCTION than in the case of thruster for DP systems and tugs. The manoeuvring requirements due to space Azimuth drives are often applied for ships requiring restrictions imply the whole range of advance higher manoeuvring capability, and are thus suitable numbers and the hull form is different from a flat for ships operating in confined waters. This is bottom barge, especially at the stern. The omni- particularly the case for inland vessels, including those directionality of the thruster units also requires some for fluvio-maritime service, called estuary vessels in attention since it increases the probability of undesired this paper. Contra-rotating Z-drives are commonly ship-thruster or thruster-thruster interactions. Another accepted as the propulsion device giving the highest important factor is the restriction of water depth for efficiency in several fields of marine industry and this type of vessels which might cause additional their efficiency has made them very attractive also for effects to the units. several types of DP-capable offshore vessels in the North Sea [1]. To the authors’ best knowledge, despite the work of Stettler [4], to date there is no literature concerning the Vessel geometry, manoeuvring capabilities and power influence of azimuth thrusters on the manoeuvring supply are main issues in the design of a propulsive performance for estuary vessels. Thus, to investigate system for estuary vessels. To meet these the manoeuvrability performance of an estuary vessel requirements more than one propulsion unit is used. equipped with two contra-rotating azimuth drives, a This implies additional operational constraints related series of captive manoeuvring tests has been carried to thruster-thruster interaction such as blind azimuth out with a ship model in the towing tank for angles and the propeller rates in overlap conditions. manoeuvres in shallow water (co-operation Flanders 10 th International Conference on Hydrodynamics October 1-4, 2012 St. Petersburg, Russia Hydraulics Research – Ghent University) in Antwerp, Coordinate systems and Conventions Belgium. Measurements of the thruster forces were performed EXPERIMENTAL SET UP in the ship bound longitudinal and transversal components, ̀3 and ̀4 (see Fig. 1(a)). The sign of the Model tests were carried out with a 1/25 scale model azimuth angle and the drift angle is shown in Fig. 1(b) of the estuary container vessel Tripoli , operating and 1(c), respectively. between the coastal port of Zeebrugge and the hinterland via the Western Scheldt estuary, the main For convenience of the analysis of the thrusters units, particulars of which are presented in Table 1. The ship the forces ̀3 and ̀4 were decomposed in the is equipped with two Veth Z-drive rudder propellers, corresponding longitudinal and normal components as presented in Table 2. Each azimuth propulsion ͎3+ and ͎4+ , respectively (see Fig. 1(a)), for the port drive is composed of two contra-rotating propellers; a side thrusters, and ͎ and ͎ for the starboard side pusher aft and a puller fore, the latter being ducted. 3. 4. thrusters. Table 1 Main particular of the estuary vessel. Particular Value LOA (length over all) 110.0 m LPP (length between perpendiculars) 106.3 m B (beam) 17.1 m T (maximum draft) 4.5 m D (depth) 5.7 m ∇ (volume displacement) 7140.0 m3 CB (block coefficient) 0.9 xG (longitudinal position centre of gravity) -0.3 m scale 1/25 Table 2 Main particular of the counter-rotating Z-drives Particular Puller Pusher Propeller diameter 1.64 m 1.50 m Design pitch ratio 1.02 m 1.13 m Blade thickness 23 mm 21 mm Expanded blade area ratio 0.7 0.9 Propeller rotation direction Right handed Left handed Number of blades 4 5 Lateral distance between Z- 10.0 m drives Duct main characteristics Nozzle length 0.656 m Internal diameter 1.968 m Fig. 1 Conventions for ship and thruster units. External diameter 1.66 m In the present study the propellers’ advance ratio, The test program consisted of bollard pull, stationary, ̈́ = , is determined by the magnitude of the speed harmonic sway and yaw and multimodal tests; a ) vector V and not by the apparent axial velocity. This description of the tests types can be found in [9-10]. is considered as large inflow angles are experienced The following parameters were varied: ship speed, by the units and using the axial inflow could lead to drift angle, thruster rates and azimuth angles. misunderstandings of the thrusters’ performance. This Moreover, tests were also carried out at different was pointed out in [11] where the assumption of the under-keel-clearances and loading conditions (all even apparent inflow velocity was only valid for small keel), see Table 3. inflow angles or if the total advance ratio had a Table 3 Tested combinations of loading condition and gross smaller value (see [3-5]). under keel clearance (in % of draft) 4.50 m 3.78 m 2.52 m DISCUSSION AND EXPERIMENTAL RESULTS 150% 198% 346% 35% According to Stettler [4], the slipstream of the puller 20% propeller strongly interacts with the pod housing and 10% strut, producing pressure fluctuations and inclusive 10 th International Conference on Hydrodynamics October 1-4, 2012 St. Petersburg, Russia large separation effects. This turns in an unsteady important effect on ship-thruster interaction. inflow to the pusher propeller. While ship-thruster interaction is the result of the Besides that, when more than one unit is installed, installation of the units, thruster-thruster interaction is other parameters such as the location, disposition, a consequence of the omni-directionality of the rates of the thrusters and the complex astern hull form thrusters. A large number of configurations of the are main concerns of interaction between thruster- thrusters is possible, which could lead to scenarios thruster and ship-thruster. For a propulsion unit where one of the units (trailing thruster) is operating installed at the ship its ambient flow is determined by in ambient flow partially or totally influenced by the the ship’s wake being the first component of ship- slipstream of the other one (leading thruster). Fig. 2 thruster interaction. Moreover, due to the omni- shows some arrangements where thruster-thruster directionality of the units, other sources of interaction interaction occurs for the portside unit. might occur. For example, when the azimuth angle is = 180 ° the thrusters’ slipstream is directed To better understand the thruster forces developments, through the length of the ship, resulting in lower the experimental data is presented in two sections: surface pressure and local friction losses [7], and for thruster forces at bollard pull condition and thruster smaller azimuth angles, such as ≈ 150 ° or forces with nonzero ship velocity. ≈ −150 ° for the starboard side and the portside units, respectively, the skeg of the ship might have an Fig. 2 Scenarios of thruster-thruster interaction 10 th International Conference on Hydrodynamics October 1-4, 2012 St. Petersburg, Russia δ = -180° T : n = 40%, n = 40% 0° SB 0° xp PS SB Typ: n PS = 40%, n SB = 40% δ = -135° -30° δ =30° SB -30° δ =30° PS δ PS SB = -90° δ SB = -45° δ SB = 0° δ -60° 60° SB = 45° -60° 60° δ SB = 90° δ SB = 135° 1.5 2 0.5 1 0.5 1 -0.5 0 -90° 90° -90° 90° -120° 120° -120° 120° -150° 150° -150° 150° 180° 180° T : n = 80%, n = 80% δ = -180° xp PS SB 0° SB Typ: n PS = 80%, n SB = 80% 0° δ = -135° -30° δ =30° SB -30° δ =30° PS δ PS SB = -90° δ SB = -45° δ SB = 0° δ -60° 60° SB = 45° -60° 60° δ SB = 90° δ SB = 135° 9 2 3 5 7 0 1 3 -2 -1 -90° 90° -90° 90° -120° 120° -120° 120° -150° 150° -150° 150° 180° 180° 0° δ = -180° T : n = 40%, n = 80% 0° Txp: n PS = 40%, n SB = 80% SB yp PS SB δ = -135° -30° δ =30° SB -30° δ =30° PS δ PS SB = -90° δ SB = -45° δ SB = 0° δ -60° 60° SB = 45° -60° 60° δ SB = 90° δ SB = 135° 5 2 3 1 3 0 1 -1 -2 -1 -90° 90° -90° 90° -120° 120° -120° 120° -150° 150° -150° 150° 180° 180° Fig.
Details
-
File Typepdf
-
Upload Time-
-
Content LanguagesEnglish
-
Upload UserAnonymous/Not logged-in
-
File Pages8 Page
-
File Size-