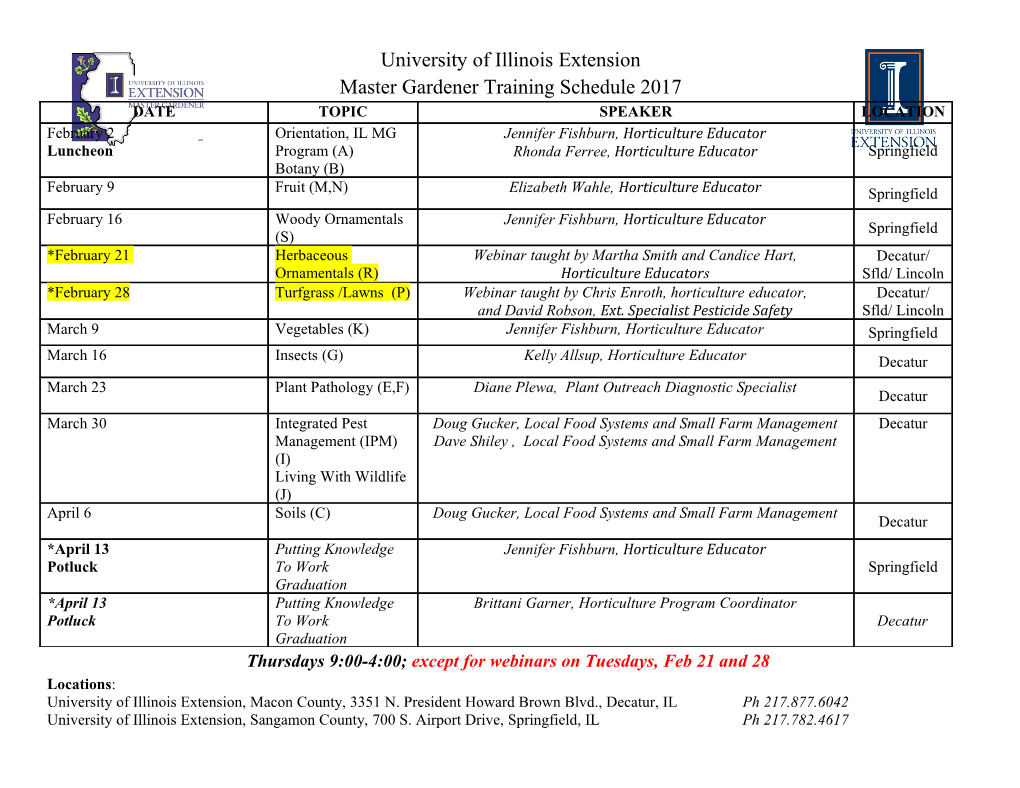
bioRxiv preprint doi: https://doi.org/10.1101/790865; this version posted February 5, 2021. The copyright holder for this preprint (which was not certified by peer review) is the author/funder, who has granted bioRxiv a license to display the preprint in perpetuity. It is made available under aCC-BY-NC-ND 4.0 International license. 1 Ancestral mitochondrial apparatus derived from the bacterial type II secretion 2 system 3 4 Lenka Horváthová1*, Vojt ch árský1*, Tomá Pánek2*$, Romain Derelle3, Jan Pyrih4, 5 Alb ta Moty ková1, Veronika Kláp ová1, Martina Vinopalová1, Lenka Marková1, 6 Lubo Voleman1, Vladimír Klime2, Markéta Petr 1, Zuzana Vaitová1, Ivan epi ka5, 7 Klára Hryzáková6, Karel Harant7, Michael W. Gray8, Mohamed Chami9, Ingrid 8 Guilvout10, Olivera Francetic10, B. Franz Lang11, estmír Vl ek12, Anastasios D. 9 Tsaousis4, Marek Eliá2#, Pavel Doleal1# 10 11 1Department of Parasitology, Faculty of Science, Charles University, BIOCEV, 12 Pr myslová 595, Vestec, 252 42, Czech Republic 13 2Department of Biology and Ecology, Faculty of Science, University of Ostrava, 14 Chittussiho 10, 710 00 Ostrava, Czech Republic 15 3School of Biosciences, University of Birmingham, Edgbaston, B15 2TT, UK 16 4Laboratory of Molecular & Evolutionary Parasitology, RAPID group, School of 17 Biosciences, University of Kent, Canterbury, CT2 7NZ, UK 18 5Department of Zoology, Faculty of Science, Charles University, Vini ná 7, Prague 2, 19 128 44, Czech Republic 20 6Department of Genetics and Microbiology, Faculty of Science, Charles University, 21 Vini ná 7, Prague 2, 128 44, Czech Republic7Proteomic core facility, Faculty of Science, 22 Charles University, BIOCEV, Pr myslová 595, Vestec, 252 42, Czech Republic 23 8Department of Biochemistry and Molecular Biology and Centre for Comparative 24 Genomics and Evolutionary Bioinformatics, Dalhousie University, Halifax, NS B3H 25 4R2, Canada 26 9Center for Cellular Imaging and NanoAnalytics, University of Basel, Mattenstrasse 26, 27 CH-4058 Basel, Switzerland 28 10Institut Pasteur, Biochemistry of Macromolecular Interactions Unit, Department of 29 Structural Biology and Chemistry, CNRS UMR3528, 75015 Paris, France 30 11Robert Cedergren Centre for Bioinformatics and Genomics, Département de Biochimie, 31 Université de Montréal, Montreal, QC, Canada H3T 1J4 32 12Institute of Molecular Genetics, Czech Academy of Sciences, 142 20 Prague 4, Czech 33 Republic 34 $Current address: Department of Zoology, Faculty of Science, Charles University, 35 Vini ná 7, Prague 2, 128 44, Czech Republic 36 37 38 39 *these authors equally contributed to the study 40 #corresponding authors: Marek Eliá ([email protected]), Pavel Doleal 41 ([email protected]) 42 43 44 45 46 bioRxiv preprint doi: https://doi.org/10.1101/790865; this version posted February 5, 2021. The copyright holder for this preprint (which was not certified by peer review) is the author/funder, who has granted bioRxiv a license to display the preprint in perpetuity. It is made available under aCC-BY-NC-ND 4.0 International license. 47 Abstract 48 The type 2 secretion system (T2SS) is present in some Gram-negative eubacteria and 49 used to secrete proteins across the outer membrane. Here we report that certain 50 representative heteroloboseans, jakobids, malawimonads and hemimastigotes 51 unexpectedly possess homologues of core T2SS components. We show that at least some 52 of them are present in mitochondria, and their behaviour in biochemical assays is 53 consistent with the presence of a mitochondrial T2SS-derived system (miT2SS). We 54 additionally identified 23 protein families co-occurring with miT2SS in eukaryotes. 55 Seven of these proteins could be directly linked to the core miT2SS by functional data 56 and/or sequence features, whereas others may represent different parts of a broader 57 functional pathway, possibly also involving the peroxisome. Its distribution in eukaryotes 58 and phylogenetic evidence together indicate that the miT2SS-centred pathway is an 59 ancestral eukaryotic trait. Our findings thus have direct implications for the functional 60 properties of the early mitochondrion. 61 62 63 Introduction 64 Mitochondria of all eukaryotes arose from the same Alphaproteobacteria-related 65 endosymbiotic bacterium1,2. New functions have been incorporated into the bacterial 66 blueprint during mitochondrial evolution, while many ancestral traits have been lost. 67 Importantly, in some cases, these losses occurred independently in different lineages of 68 eukaryotes, resulting in a patchy distribution of the respective ancestral mitochondrial 69 traits in extant eukaryotes. Examples are the ancestral mitochondrial division apparatus 70 (including homologues of bacterial Min proteins), the aerobic-type rubrerythrin system, 71 or the tmRNA-SmpB complex, each retained in different subsets of distantly related 72 protist lineages35. It is likely that additional pieces of the ancestral bacterial cell 73 physiology will be discovered in mitochondria of poorly studied eukaryotes. 74 An apparent significant difference between the mitochondrion and bacteria 75 (including those living as endosymbionts of eukaryotes) lies in the directionality of 76 protein transport across their envelope. All bacteria export specific proteins from the cell 77 via the plasma membrane using the Sec or Tat machineries6, and many diderm (Gram- 78 negative) bacteria exhibit specialized systems mediating further protein translocation 79 across the outer membrane (OM)7. In contrast, the mitochondrion depends on a newly 80 evolved protein import system spanning both envelope membranes and enabling import 81 of proteins encoded by the nuclear genome8. The capacity of mitochondria to secrete 82 proteins seems to be limited. Mitochondrial homologues of Tat translocase subunits occur 83 in some eukaryotic taxa, but their role in protein secretion has not been established9,10. A 84 mitochondrial homologue of the SecY protein (a Sec translocase subunit) has been 85 described only in jakobids11,12 and its function remains elusive13. No dedicated machinery 86 for protein export from the mitochondrion across the outer mitochondrial membrane has 87 been described. 88 One of the best characterized bacterial protein translocation machineries is the so- 89 called type 2 secretion system (T2SS)14,15. The T2SS belongs to a large bacterial 90 superfamily of type 4 pili (T4P)-related molecular machines, most of which secrete long 91 extracellular filaments (pili) for motility, adhesion, or DNA uptake1618. The T2SS 92 constitutes a specialized secretion apparatus, whose filament (pseudopilus) remains in the bioRxiv preprint doi: https://doi.org/10.1101/790865; this version posted February 5, 2021. The copyright holder for this preprint (which was not certified by peer review) is the author/funder, who has granted bioRxiv a license to display the preprint in perpetuity. It is made available under aCC-BY-NC-ND 4.0 International license. 93 periplasm14,15. It is composed of 12-15 conserved components, commonly referred to as 94 general secretion pathway (Gsp) proteins, which assemble into four main subcomplexes 95 (Fig. 1A). The OM pore is formed by an oligomer of 15-16 molecules of the GspD 96 protein19,20. The subcomplex in the inner membrane (IM) is called the assembly platform 97 and consists of the central polytopic membrane protein GspF surrounded by single-pass 98 membrane proteins GspC, GspL, and GspM21. GspC links the assembly platform to the 99 OM pore by interacting with the periplasmic N-terminal domain of GspD22,23. The third 100 subcomplex, called the pseudopilus, is a helical filament formed mainly of GspG 101 subunits, with minor pseudopilins (GspH, GspI, GspJ and GspK) assembled at its tip24. 102 Pseudopilus assembly from its inner membrane base is believed to push the periplasmic 103 T2SS substrate through the OM pore. The energy for pseudopilus assembly is provided 104 by the fourth subcomplex, the hexameric ATPase GspE, interacting with the assembly 105 platform from the cytoplasmic side25,26. Substrates for T2SS-mediated secretion are first 106 transported by the Tat (as folded proteins) or the Sec (in an unfolded form) system across 107 the IM into the periplasm, where they undergo maturation and/or folding. The folded 108 substrates are finally loaded onto the pseudopilus for the release outside the cell via the 109 OM pore. The known T2SS substrates differ among taxa and share no common sequence 110 or structural features. Proteins transported by the T2SS in different species include 111 catabolic enzymes (such as lipases, proteases or phosphatases) and, in the case of 112 bacterial pathogens, toxins14. A recent survey of bacterial genomes showed that the T2SS 113 is mainly present in Proteobacteria18. Crucially, neither the T2SS nor other systems of the 114 T4P superfamily have been reported from eukaryotes7,14,18. 115 Here we show that certain distantly related eukaryotes unexpectedly contain 116 homologues of key T2SS subunits representing all four functional T2SS subcomplexes. 117 We provide evidence for mitochondrial localization of some of these eukaryotic Gsp 118 protein homologues and describe experimental results supporting the idea that they 119 constitute a system similar to the bacterial T2SS. Furthermore, we point to the existence 120 of 23 proteins with a perfect taxonomic co-occurrence with the eukaryotic Gsp 121 homologues. Some of these co-occurring proteins seem to be additional components of 122 the mitochondrial T2SS-related machinery, whereas
Details
-
File Typepdf
-
Upload Time-
-
Content LanguagesEnglish
-
Upload UserAnonymous/Not logged-in
-
File Pages42 Page
-
File Size-