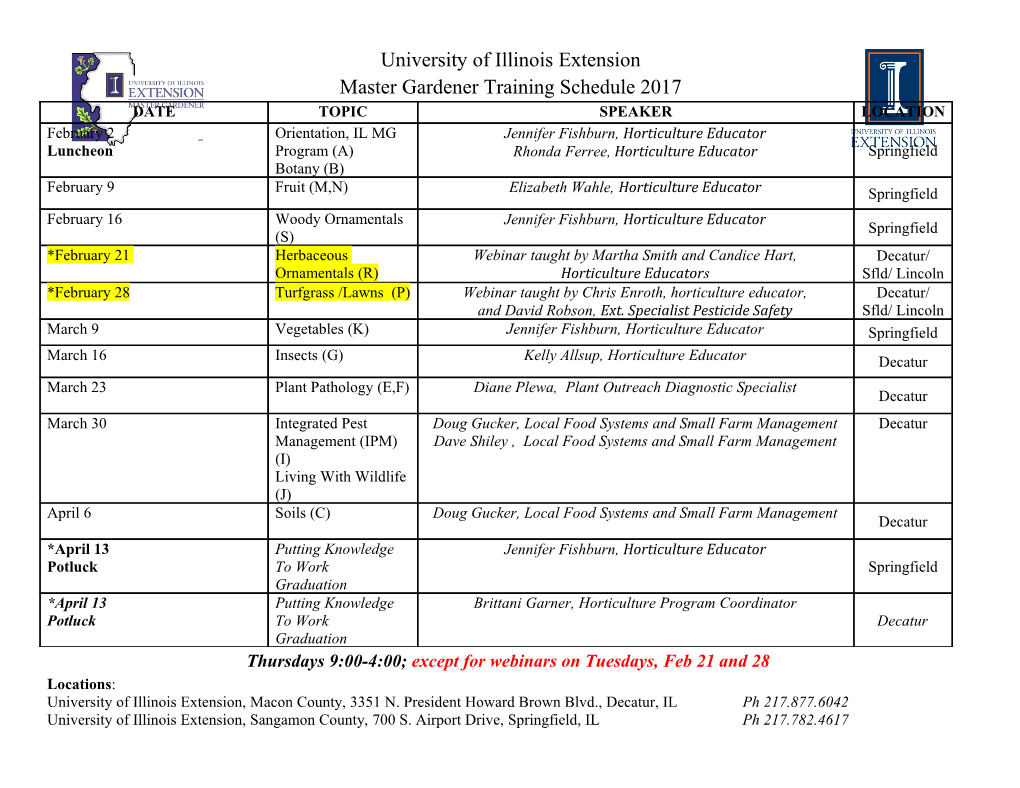
Environ. Sci. Technol. 2008, 42, 3349–3355 real groundwater aquifers, and may provide transport distances Ionic Strength and Composition of 10s to 100s of meters in unconsolidated sandy aquifers at Affect the Mobility of injection velocities used for emplacement. 0 Surface-Modified Fe Nanoparticles Introduction in Water-Saturated Sand Columns Nanoscale zerovalent iron (NZVI) has been evaluated as an in situ treatment alternative for chlorinated solvent non- NAVID SALEH,† HYE-JIN KIM,† aqueous phase liquid (NAPL) source zones (1-3). Laboratory TANAPON PHENRAT,† studies (4, 5) and field pilot studies (2) have demonstrated KRZYSZTOF MATYJASZEWSKI,§ low mobility of NZVI in porous media and the challenges in | ROBERT D. TILTON,‡, AND distributing NZVI evenly in the subsurface. Inhibiting NZVI GREGORY V. LOWRY*,†,‡ aggregation and the attachment of NZVI to aquifer grains is Department of Civil and Environmental Engineering, essential for making them mobile (5). Enhanced mobility of Department of Chemical Engineering, Department of iron nanoparticles was demonstrated by Schrick et al. (4) Chemistry, and Department of Biomedical who used an anionic hydrophilic carbon and poly(acrylic Engineering, Carnegie Mellon University, acid) as supports for NZVI and by He et al. (6) who used Pittsburgh, Pennsylvania 15213-3890 carboxymethyl cellulose to surface modify Fe-Pd nanopar- ticles. Saleh et al. (5) demonstrated that polyelectrolytes and Received August 02, 2007. Revised manuscript received surfactants adsorbed directly to NZVI can stabilize NZVI December 13, 2007. Accepted January 22, 2008. dispersions, decrease attachment to silica surfaces, and thereby enhance NZVI mobility in porous media while maintaining good reactivity. Surface modification of nanoparticles using charged The surfaces of nanoscale zerovalent iron (NZVI) used for polymers (polyelectrolytes) or surfactants (3, 7) increases groundwater remediation must be modified to be mobile in the the surface charge of the particles and provides electrostatic subsurface for emplacement. Adsorbed polymers and double layer (EDL) repulsions between particles to inhibit surfactants can electrostatically, sterically, or electrosterically aggregation, and between particles and surfaces to inhibit stabilize nanoparticle suspensions in water, but their efficacy attachment. For particles that are only electrostatically will depend on groundwater ionic strength and cation type as stabilized, changes in ionic strength and ionic composition well as physical and chemical heterogeneities of the aquifer can change the EDL screening length, i.e., the range and magnitude of repulsive double layer interaction between material. Here, the effect of ionic strength and cation type on the particles, or between particles and surfaces, and thus change mobility of bare, polymer-, and surfactant-modified NZVI is their stability (resistance to aggregation) and mobility in the evaluated in water-saturated sand columns at low particle subsurface (8-11). In the case of polyelectrolyte surface concentrations where filtration theory is applicable. NZVI surface coatings, so-called electrosteric repulsions also arise between modifiers include a high molecular weight (MW) (125 kg/mol) particles and between particles and surfaces that are non- poly(methacrylic acid)-b-(methyl methacrylate)-b-(styrene adsorbing for the polyelectrolyte. Electrosteric repulsions sulfonate) triblock copolymer (PMAA-PMMA-PSS), polyaspartate tend to be quite strong and long-ranged, and are known to which is a low MW (2-3 kg/mol) biopolymer, and the be robust even at ionic strengths on the order of several surfactant sodium dodecyl benzene sulfonate (SDBS, MW ) hundred mM where electrostatic double layer repulsions are highly screened (12, 13). Both electrostatic and electrosteric 348.5 g/mol). Bare NZVI with an apparent -potential of -30 ( repulsions can help to decrease NZVI aggregation and 3 mV was immobile. Polyaspartate-modified nanoiron (MRNIP) attachment. - ( with an apparent -potential of 39 1 mV was mobile at Reported studies on the dispersion stability and mobility + 2+ low ionic strengths (<40 mM for Na and <0.5 mM for Ca ), of NZVI in porous media have used either deionized water and had a critical deposition concentration (CDC) of ∼770 or water at very low ionic strength (4-6) that is not mM Na+ and ∼4 mM for Ca2+. SDBS-modified NZVI with a representative of groundwater conditions. In groundwater, similar apparent -potential (-38.3 ( 0.9 mV) showed similar the concentration of monovalent cations (e.g., Na+,K+)is + + behavior (CDC ∼350 mM for Na+ and ∼3.5 mM for Ca2+). Triblock typically 1-10 mM and divalent cations, e.g., Ca2 ,Mg2 is - copolymer-modified NZVI had the highest apparent -potential typically 0.1 2mM(14, 15). Although the groundwater ionic (-50 ( 1.2 mV), the greatest mobility in porous media, and strength and ionic composition are likely to have a significant a CDC of ∼4 M for Na+ and ∼100s of mM for Ca2+. The high effect on the subsurface mobility of surface-modified NZVI, a systematic investigation of these effects has not been mobility and CDC is attributed to the electrosteric stabilization reported. A fundamental understanding of the effect of ionic afforded by the triblock copolymer but not the other modifiers strength and composition for different surface-modified which provide primarily electrostatic stabilization. Thus, particles can be used to estimate a travel distance based on electrosteric stabilization provides the best resistance to the modifier type and on the site geochemistry, and thus changing electrolyte conditions likely to be encountered in could enable controlled in situ placement. The objectives of this study are to (1) quantify NZVI mobility enhancement from three different classes of NZVI surface modifiers including a low molecular weight (MW) * Corresponding author e-mail: [email protected]. surfactant (sodium dodecyl benzene sulfonate), a high MW † Department of Civil & Environmental Engineering. ‡ Department of Chemical Engineering. (125 kg/mol) triblock copolymer with a poly(methacrylic § Department of Chemistry. acid)-b-poly(methyl-methacrylate)-b-poly-(styrene sulfonate) | Department of Biomedical Engineering. (PMAA-PMMA-PSS) architecture (3), and a moderate MW 10.1021/es071936b CCC: $40.75 2008 American Chemical Society VOL. 42, NO. 9, 2008 / ENVIRONMENTAL SCIENCE & TECHNOLOGY 9 3349 Published on Web 04/05/2008 (2-3 kg/mol) polypeptide (polyaspartate); and (2) to quantify exponent describes the sensitivity of the particles to an the sensitivity of each type of coating to changes in ionic increase in salt concentration. The CDC and are determined strength and composition. Surface-modified reactive nanoiron from a plot of log R (determined in column experiments at particles (RNIP) are prepared, and the apparent -potential varying Cs)vslogCs. A higher CDC indicates a higher and transport of each in saturated sand columns is deter- resistance to salt-induced particle attachment (17), i.e. a mined as a function of [Na+] and [Ca2+]. A single-collector higher CDC indicates that the modifier does a better job at efficiency model (16) is used to calculate the “sticking mitigating attachment. Particles that are only electrostatically coefficient”, R, from breakthrough data collected for each stabilized would be expected to be vulnerable to deposition modifier type in columns, and using quartz crystal mi- at ionic strengths in the tens to hundreds of mM range. Data crobalance with dissipation (QCMD-D) at varying ionic collected by the quartz crystal microbalance (QCMD-D) in strength and composition. An empirical equation is used to a flow through cell are analyzed similarly to determine the determine the critical deposition concentration (CDC) and CDC and (16). sensitivity to changes in salt concentration for each modifier Using R determined in column studies and the column (17). Finally, DLVO calculations are used to postulate reasons properties (e.g., dc, , ηo), the filtration or travel length required for the observed mobility enhancement of each modifier. to remove 99% of the particles, i.e., where CL/C0 ) 0.01 is calculated using eq 5 (18, 20). The choice of CL/C0 is arbitrary, Theory but is the same for each particle evaluated. Particle Attachment and Quantifying Relative Transport 2d Distances. Using the single collector efficiency model (16, 18) )- c LT ln(CL ⁄ C0)( - R ) (5) a dimensionless deposition rate η0 can be calculated using 3(1 ε) η0 eq 1, where ηD, ηI, and ηG are collector efficiency components for particle transport to the collector due to diffusion, Particle-Collector Interaction Energy Calculation. Par- interception, and gravity, respectively (16). ticle-collector electrostatic interaction energies are calculated using classical DLVO theory (21) for sphere-flat plate ) + + η0 ηD ηI ηG (1) interaction, i.e., assuming the particles are uniform spheres and small relative to the sand grains. For Ca2+ (added as Essentially, η0 is the probability that a particle will collide CaCl2), a symmetric electrolyte condition was assumed for with the collector grain through one of the three modes of simplicity. The maximum error from this assumption is 10%, transport (diffusion, interception, and transport), accounting even at very high salt concentrations (22). Details of the for system hydrodynamics, particle size, density, and van equations and parameters used in these calculations are der Waal’s forces. For nanoparticles of the size and density provided in Supporting Information. The surface potential
Details
-
File Typepdf
-
Upload Time-
-
Content LanguagesEnglish
-
Upload UserAnonymous/Not logged-in
-
File Pages7 Page
-
File Size-