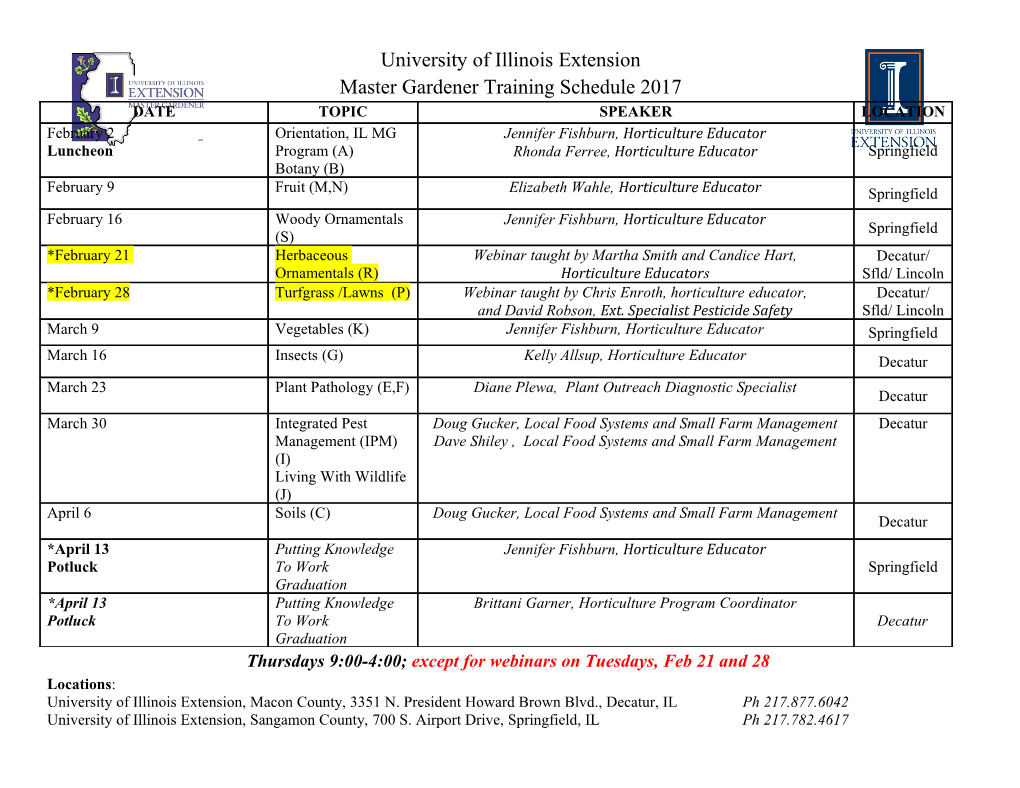
Technische Universität München Max-Planck-Institut für Astrophysik Progenitor systems of Type Ia Supernovae: mergers of white dwarfs and constraints on hydrogen-accreting white dwarfs Rüdiger M. Pakmor Vollständiger Abdruck der von der Fakultät für Physik der Technischen Universität Mün- chen zur Erlangung des akademischen Grades eines Doktors der Naturwissenschaen (Dr. rer. nat.) genehmigten Dissertation. Vorsitzender: Univ.-Prof. Dr. R. Krücken Prüfer der Dissertation: Õ. Priv.-Doz. Dr. F. K. Röpke ó. Univ.-Prof. Dr. H. Friedrich Die Dissertation wurde am ÕÉ.þß.óþÕþ bei der Technischen Universität München eingereicht und durch die Fakultät für Physik am Õ¦.Õó.óþÕþ angenommen. Contents 1 Introduction 1 2 Type Ia supernovae 5 ö.× Observational properties . .£ ö.×.× Classication . .£ ö.×.ö Lightcurves . .å ö.×.î Spectra . .Ì ö.×.® Subclasses . .Ì ö.×.£ Polarization . ×× ö.ö eoretical description . ×× ö.ö.× Progenitor systems . ×ö ö.ö.ö Explosion scenarios . ×à 3 The Dynamic Merger Scenario 23 î.× eScenario...................................... öî î.ö Modeling pipeline . öî î.î Model parameters . öå î.® e merger of two ý.ÀM⊙ whitedwarfs...................... öÌ î.®.× Setup . öÌ î.®.ö Inspiral and merger . öÌ î.®.î Formation of the detonation . î× î.®.® Explosion . î® î.®.£ Nucleosynthesis . îà î.®.å Comparison with observations . îÌ î.®.à Changing the mass ratio . ®® î.£ Dark explosions from low-mass mergers . ®å î.å Normal type Ia supernovae from mergers of white dwarfs . ®Ì î.à Superluminous type Ia supernovae: mergers of the most massive CO white dwarfs? ......................................... £ö î.à.× Modeling the merger . ££ î.à.ö e explosion . £å î.à.î Comparison with Observations . £Ì î. Summary of the Dynamic Merger Model . å× 4 The impact of type Ia supernova ejecta on their companions 63 ®.× Observational and theoretical constraints . åî i Contents ®.ö Modeling approach . å® ®.î Tests of implementation . å£ ®.® Parameter studies . åÌ ®.®.× Explosion energy . àÿ ®.®.ö Distance . àö ®.®.î Companion star . àö ®.£ Observational implications . ࣠®.£.× Hydrogen detection in SN Ia spectra . ࣠®.£.ö Hole in the ejecta . ࣠®.£.î Identifying companion stars in supernova remnants . àà ®.å Massive main sequence companion stars . à 5 Codes 81 £.× Stellar Gadget . × £.×.× Energy equation . ö £.×.ö Gravitational soening . î £.×.î Equation of state . î £.×.® Nuclear reaction network . ® £.×.£ Wakeup mechanism . £ £.×.å Initial conditions . å £.×.à Relaxation . £.ö LEAFS . Ìÿ £.î Mapping ........................................ Ì× £.î.× Grid to particles . Ìö £.î.ö Particles to grid . Ìö 6 Summary 97 ii List of Figures ×.× Supernova ÕÉɦD in the outskirts of disk galaxy NGC ¦¢óä. (Credit: NASA)ö ö.× Supernova classication scheme . .£ ö.ö Lightcurves of SN óþþìdu . .à ö.î Phillips relation . î.× ý.ÀM⊙ ý.ÀM⊙ merger: density . îÿ î.ö ý.ÀM⊙ ý.ÀM⊙ merger: temperature . î× î.î ý.ÀM⊙ + ý.ÀM⊙ merger: density vs. temperature . îî î.® ý.ÀM⊙ + ý.ÀýM⊙ merger: resolution study . î® î.£ ý.ÀM⊙ + ý.ÀM⊙ merger: explosion . î£ î.å ý.ÀM⊙ + ý.ÀM⊙ merger: mass and abundance prole . îå î.à ý.ÀM⊙ + ý.ÀM⊙ merger: ejecta composition . îà î. ý.ÀM⊙ + ý.ÀM⊙ merger: lightcurve . ®ÿ î.Ì ý.ÀM⊙ + ý.ÀM⊙ merger: maximum light spectrum . ®× î.×ÿ Mass ratio+ . ®£ î.×× ý.M⊙ +ý.M⊙ merger: density vs. temperature . ®å î.×ö ý.M⊙ ý.M⊙ merger: explosion . ®à î.×î ý.M⊙ + ý.M⊙ merger: ejecta composition . ® î.×® mergers+ of two Ô.ýM⊙ and two Ô.ÔM⊙: density vs. temperature . ®Ì î.×£ Ô.ýM⊙ +Ô.ýM⊙ merger: explosion . £× î.×å Ô.ýM⊙ Ô.ýM⊙ merger: explosion . £× î.×à Composition+ in velocity space from the explosion of a merger of two equally massive+ carbon/oxygen white dwarfs . £ö î.× Ô.ýM⊙ Ô.òM⊙ merger: density . £® î.×Ì Ô.ýM⊙ Ô.òM⊙ merger: temperature . £® î.öÿ Ô.ýM⊙ + Ô.òM⊙ merger: density vs temperature . ££ î.ö× Ô.ýM⊙ + Ô.òM⊙ merger: explosion . £å î.öö Ô.ýM⊙ + Ô.òM⊙ merger: ejecta composition . £à î.öî Ô.ýM⊙ + Ô.òM⊙ merger: bolometric lightcurve . £Ì + ®.× Snapshots+ of the evolution of the companion star in the HCV scenario . åå ®.ö Mass loss of the companion star depending on the time aer the supernova explosion for dierent resolutions. å ®.î Stripped mass for dierent supernova energies in model rpì_óþa. e dashed line corresponds to the t shown in the plot. à× iii List of Figures ®.® Stripped mass versus binary separation for model rpì_óþa. àî ®.£ Comparison of density proles of the companion star in model rpì_óþa at the time of the explosion and a single star with the same mass and nuclear age. ........................................... à® ®.å Snapshots of the evolution of the supernova ejecta in the rpì_óþa scenario . àå ®.à Snapshot of simulation rpì_óþa taken ýýý s aer the explosion . àà ®. e impact of the ejecta of a type Ia supernova on a massive main sequence companionstar .................................... àÌ £.× Comparison of dierent methods to create initial conditions . Ì iv List of Tables î.× Merger models . ö î.ö ý.ÀM⊙ ý.ÀM⊙ merger: nucleosynthesis yields . î î.î List of analyses of superluminous type Ia supernovae published so far . £î î.® Ô.ýM⊙ +Ô.òM⊙ merger: nucleosynthesis yields . £ ®.× Resolution+ test . åÌ ®.ö Parameters of the progenitor models . åÌ v 1 Introduction Most objects on the sky appear static when observed at human timescales, as they evolve on timescales of millions to billions of years. us, we will never be able to observe the evolution of a star or a galaxy directly. So we have to observe most objects as the are and try to infer their past and future by comparison with other, similar but newer and older objects. ere are, however, objects that appear and disappear again on timescales of days, months or years. Supernovae are among the most spectacular ones and humans have noticed and described them already a thousand years ago. If they are located in the Milky Way they appear as luminous very bright stars on the sky. Some of the most famous historical su- pernovae, e.g. SN Õþþä and SN Õþ¢¦ have been observed and documented by astronomers on dierent continents providing an opportunity to historians to synchronize calendars of dierent civilizations. Supernovae are magnicent events. eir properties are extreme in every sense. Within a second, they release a staggering amount of energy, similar to the energy the sun produces over its whole lifetime. is energy release causes a powerful explosion, that ejects material of the order of the mass of the sun at velocities up to tens of thousands kilometers per second. A small fraction of this energy is released in the form of light and powers the visual display of the supernova. Figure Õ.Õ shows supernova SN ÕÉɦD in the outskirts of disk galaxy NGC ¦¢óä. e supernova is as bright as the center of the galaxy. In the explosion itself temperatures of several billion Kelvin are reached that lead to explosive nucleosynthe- sis. is way, supernovae have produced virtually all heavy elements and thus shaped the Universe. As supernovae are subject to such extremes, they probe physical conditions that are not accessible on Earth. While this enables us to use them to learn about the laws of Nature in regimes we could not test otherwise, it also makes it very challenging to understand super- novae from a theoretical point of view. Since there are many dierent physical processes Õ Õ Introduction Figure Õ.Õ: Supernova ÕÉɦD in the outskirts of disk galaxy NGC ¦¢óä. (Credit: NASA) involved that interact with each other in very complex ways, supernovae elude any simple theoretical description. However, it is possible to use numerical models which incorporate the relevant physics to try to understand the properties of supernovae we observe. In principle they allow to follow the evolution of complex systems if we know the initial state of the system and model the physical processes involved adequately. To model supernovae, it is necessary to account for many dierent areas of physics including hydrodynamics, nuclear reactions, equations of state of extremely dense and ar- bitrarily degenerate matter, radiative transfer, and many more. Unfortunately, some of these are not completely understood themselves, let alone their combination. At the same time, it is not even feasible to model all physical processes as good as possible in a numerical model that includes all of them due to constrains in computational resources. us, to understand a supernova in which processes of dierent physical areas act at the same time in numerical models we have to use approximations to the basic laws of Nature. In addition, we do not know the initial conditions from which supernovae arise in detail. Nevertheless, as shown in this thesis, we are able to understand qualitatively how some of these events work and even do quantitative tests of our models. Beside being fascinating objects in their own right, supernovae are directly connected to several other elds of astrophysics. ey play an important role in the evolution of galaxies and they are the key to the chemical evolution of the Universe. Supernovae of ó type Ia, a specic species of supernovae,.
Details
-
File Typepdf
-
Upload Time-
-
Content LanguagesEnglish
-
Upload UserAnonymous/Not logged-in
-
File Pages117 Page
-
File Size-