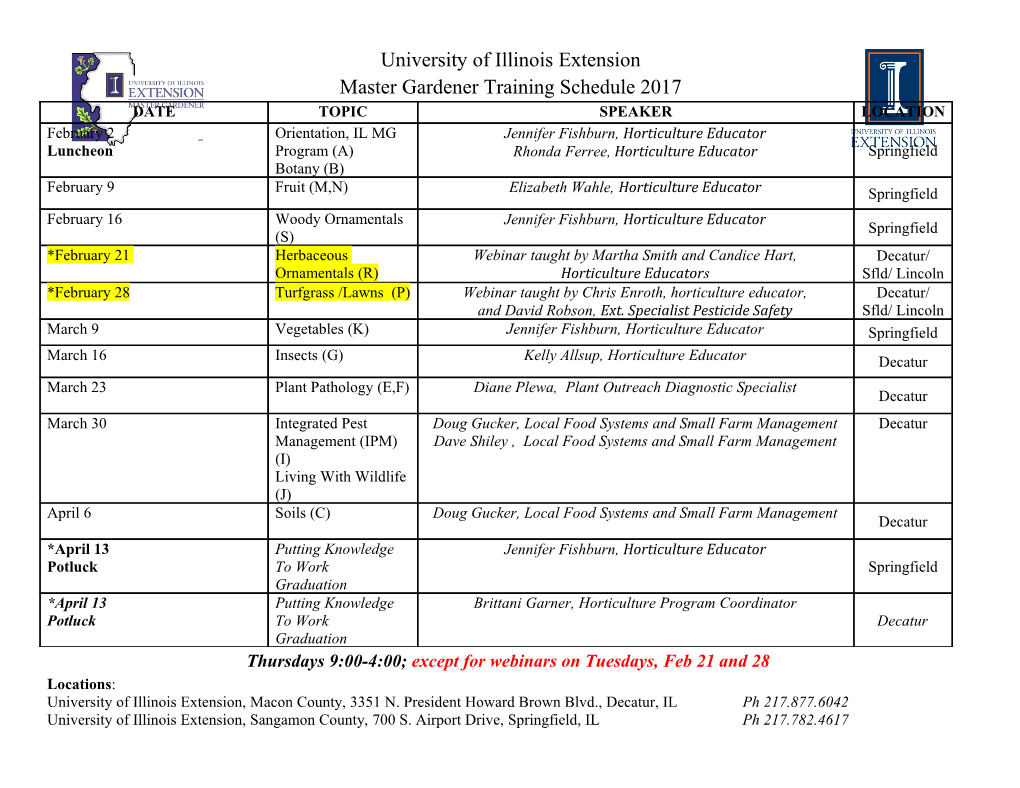
PHYSICAL REVIEW APPLIED 16, 014059 (2021) Arbitrarily Large Neutron Amplification in Subcritical Nuclear Reactors Antoine Tilloy * Max-Planck-Institut für Quantenoptik, Hans-Kopfermann-Straße 1, Garching 85748, Germany (Received 13 December 2020; revised 11 June 2021; accepted 22 June 2021; published 26 July 2021) In a subcritical reactor, each neutron produces only keff < 1 neutrons per generation (asymptotically and on average), and thus, the neutron population decreases exponentially in the absence of an external source. The chain reaction is thus easy to stop, making such reactors inherently stable. Interestingly, and contrary to common wisdom, there is no relation between keff, the external source intensity, and the output power of the reactor. Here, various possible strategies to exploit this fact are presented, and they are applied to the design of a rudimentary multilayer system that allows an arbitrarily large number of fissions per source neutron to be reached, while keeping keff < 1fixed(here,keff = 0.97). The behavior of the amplifier is verified with simple Monte-Carlo transport simulations. The proposal admittedly brings complications and subtleties that need to be studied further, but, if developed, could allow subcritical reactors to be driven with faint neutron sources, e.g., radioisotope based. DOI: 10.1103/PhysRevApplied.16.014059 I. INTRODUCTION source, it can give the wrong order of magnitude in more complicated situations. Subcritical nuclear reactors are serious candidates for We need to keep track of neutron generations and con- the incineration of minor actinides produced by current sider the fact that the multiplication per neutron, k , can light-water reactors. With an asymptotic neutron multipli- i be generation dependent because of the geometry of the cation, k < 1, they allow an intrinsically stable operation eff system. The number of neutrons produced from a single- without the need for delayed neutrons and can thus oper- source neutron is, on average, ate with a much higher minor actinide content than more standard critical reactors. However, neutrons have to come +∞ i 1 from somewhere, and one [1] of the drawbacks of such N = k + k k + k k k +···= k def.= . 1 1 2 1 2 3 j 1 − k designs lies in the need for an efficient external neutron i=1 j =1 s source. Current proposals use a spallation source driven by (1) a high-current proton accelerator. To reach an industrially meaningful actinide incineration or electricity production, This N, or equivalently ks, is what we focus on when the accelerator power required goes well beyond what is we consider the total neutron multiplication of the sys- available off-the-shelf. tem and the output power for a given source. ks is the The high power need comes from the requirement of equivalent multiplication we would need to get the same a keff value that is not too close to 1.0, to ensure safe N had the multiplication ki been generation independent. operation; the choice of keff 0.95 is usually made in the Importantly, it is unrelated to keff, which is defined as literature [2]. At an intuitive level, this suggests that one def. neutron can produce, on average, 0.95 neutrons per gener- keff = lim ki,(2) ation, and thus, a number of fissions in the order of 20 from i→+∞ the first fission induced by the spallation source. While which is the asymptotic multiplication. Only keff governs this rule of thumb may give a correct order of magnitude the long-term reactor kinetics and is thus relevant to assess for standard accelerator-driven subcritical reactor (ADSR) criticality. We see that, in principle, there is no theoretical designs and explains the need for a high-intensity neutron obstacle to an arbitrarily large N (ks arbitrarily close to 1.0) with a fixed subcritical keff, if the initial ki values are large. *[email protected] In fact, some ADSR designs obtain modest amplifi- cation from the naive keff = ks relation, with a highly Published by the American Physical Society under the terms of enriched core near the spallation source surrounded by the Creative Commons Attribution 4.0 International license. Fur- ther distribution of this work must maintain attribution to the low-enriched blankets. However, such designs typically author(s) and the published article’s title, journal citation, and reduce the accelerator requirements only by a moderate DOI. Open access publication funded by the Max Planck Society. amount. 2331-7019/21/16(1)/014059(6) 014059-1 Published by the American Physical Society ANTOINE TILLOY PHYS. REV. APPLIED 16, 014059 (2021) This raises a natural question: is it possible to design a core such that keff is fixed but N is large enough to sub- stantially reduce the accelerator requirements? Can mul- tiplication be increased to the point that a much smaller accelerator can be used or even that much weaker radioiso- tope sources can be used? Is there a physical limit to (a) (d) amplification due to neutron transport in existing materi- als? Is there a practical limit with current technology and engineering constraints? (b) II. STRATEGIES To increase N, while keeping keff < 1, one needs to create an irreversibility or decoupling in the neutron cur- rent. Imagine we are given a perfect neutron diode, that (c) is, a material letting neutrons go in one, and only one, direction. Then, we could easily stack subcritical sections, separating them by the diodes and the total would remain subcritical, with the same keff. However, neutron amplifi- cation would be enhanced geometrically with the number FIG. 1. Strategies for multistage amplification. Subcritical cells separated by ideal neutron diodes act as neutron amplifiers. of layers, without obvious physical limit. In practice, this can be implemented with four distinct physi- Of course, perfect neutron diodes do not grow on trees, cal strategies: (a) thermal capture, (b) fast capture, (c) threshold and the challenge lies in creating a genuine unidirectional- fission, and (d) geometry. ity of the neutron current, so that the criticality, keff, does not increase (or only in a subleading way) when stacking more subcritical layers. neutrons. As before, this allows a one-way amplifier to be There are at least four conceptually different ways to cre- constructed with the addition of a moderator. ate a one-way neutron current between different parts of a The fourth option [Fig. 1(d)] is purely geometric and is, reactor, as summarized in Fig. 1. In practice, these differ- in a way, the simplest (see Ref. [2]). The idea is to decou- ent methods can be combined and are only separated to ple stages by having them grow in size (or in surface) as emphasize the physical mechanism at play. one moves away from the source. This way, neutrons from The strategy outlined in Fig. 1(a) corresponds to one that an earlier stage can reach the following one, where they was considered as early as 1957 [3]. The idea is to exploit induce fission, but this fission creates neutrons that have the fact that certain materials, like cadmium, are almost less chance to hit the initial stage. An example consists of transparent to fast neutrons, but have a large capture cross considering concentric fissile spheres of quickly growing section for thermal neutrons. Intuitively, by putting such radii and shrinking width. If the ratio of the radii is large a thermal absorber and following it with a moderator, one enough, the total keff of the set of spheres is the same as that obtains a material that can be (approximately) crossed only of each of them taken individually. However, the number one way by neutrons (and converts fast neutrons into slow of fissions is amplified at each layer. neutrons in the forward direction). Finally, a more recent idea is to exploit magnetic fields The second strategy [Fig. 1(b)] is, in a way, opposite to deflect neutrons via coupling to their spin [4]. As it is far to the first. Instead of capturing slow neutrons, one could more speculative than the previous strategies outlined, this selectively capture fast neutrons with a material like low- option is not considered further here. enriched uranium, which works as a neutron multiplier only in the thermal spectrum, while neutron capture by III. PREAMPLIFIER EXPLORATION 238U proportionally dominates in a fast spectrum. The idea is to put a moderator first and then this fast-spectrum cap- The objective of this study is to see how a combination ture material to obtain a diode. This one-way amplification of the previous strategies could fare with physically realis- through thermal fission is likely to be insufficient to create tic materials and geometry (including neutron loss). To this genuine irreversibility alone, but seems to play a role in end, it seems easier to distinguish between two levels of multiplication achieved by various proposals. amplification that would be joined together in a complete The third option [Fig. 1(c)] is to use a threshold fis- reactor, but that come with starkly different constraints. sile material like 237Np or 241Am, which is only fissile The first stage, the preamplifier, would bring an arbitrarily in a fast spectrum and, consequently, multiplies only fast faint neutron flux to the intensity needed to drive a second 014059-2 ARBITRARILY LARGE NEUTRON... PHYS. REV. APPLIED 16, 014059 (2021) “power” stage. This second stage, a more standard reactor core, would be typically made of only a few simple layers, with low amplification but high power density. A proper study of a modestly amplifying reactor core is difficult, but is reported in the literature [5,6]. In fact, such designs are even implemented (at zero power) in the YALINA-Booster experiment at The Joint Institute for Power and Nuclear Research in Belarus [7–9].
Details
-
File Typepdf
-
Upload Time-
-
Content LanguagesEnglish
-
Upload UserAnonymous/Not logged-in
-
File Pages6 Page
-
File Size-