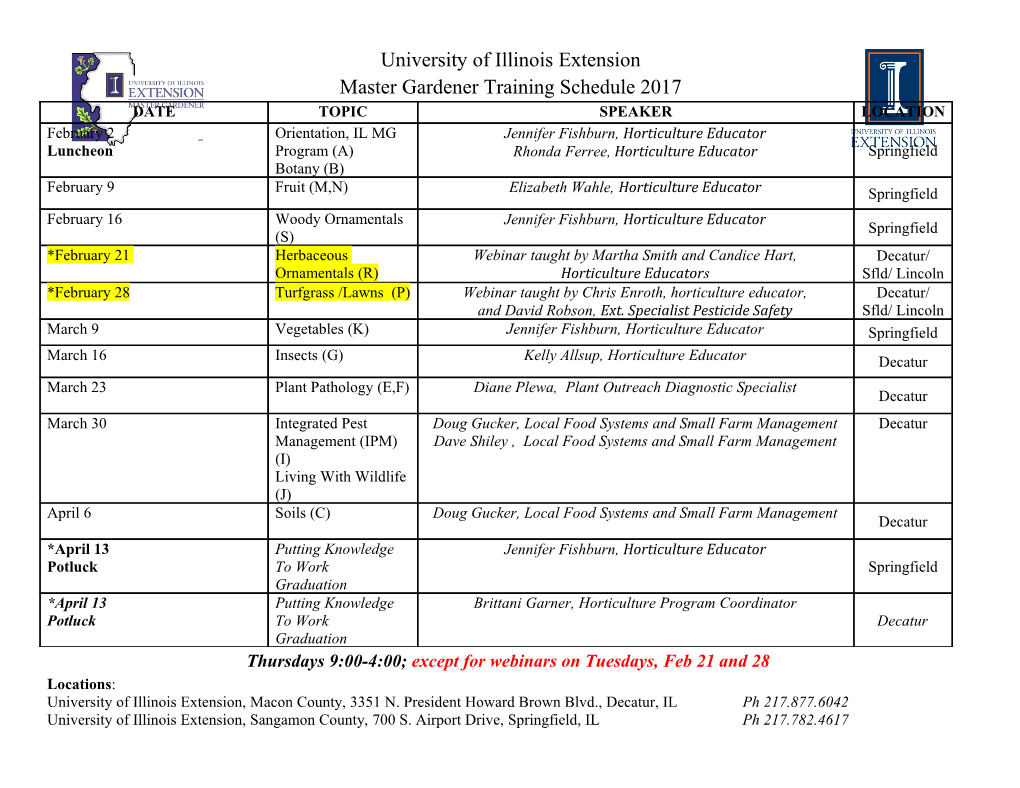
Department of Astronomy Licentiate thesis Exploring the Diagnostic Value of He i D3 in the Solar Chromosphere Tine Libbrecht October, 2016 Supervisors Jaime de la Cruz Rodríguez Göran Scharmer Jorrit Leenaarts Tine Libbrecht Exploring the Diagnostic Value of He I D3 in the Solar Chromosphere Licentiate thesis, October, 2016 Supervisors: Jaime de la Cruz Rodríguez, Göran Scharmer and Jorrit Leenaarts Stockholm University Institute for Solar Physics Department of Astronomy AlbaNova University Center SE - 106 91 and Stockholm Abstract This thesis focusses on observations of the He I D3 line at 5876 Å in the solar chromosphere. The He I D3 line is part of the triplet system of neutral helium (as is the more commonly observed He I 10830 Å line). The triplet levels in helium are thought to be populated through a photoionization-recombination mechanism. In active regions, helium is ionized by EUV photons from the transition region and corona which are backscattered towards the chromosphere. Electrons can recombine in both singlet and triplet states in neutral helium, giving rise to opacity in He I D3 and He I 10830 Å. Due to the line formation mechanism and sensitivity to magnetic fields, the He I D3 line is a useful tool to measure magnetic fields and to study reconnection events and heating in the chromosphere. Observations of the He I D3 line are challenging - especially on the solar disk - because the line is generally weak and entirely absent outside active regions. In the last decade, the limits of high-resolution imaging and spectro-polarimetry have been pushed by advances in adaptive optics and instrumentation. High-resolution imaging and spectro-polarimetry of He I D3 have revealed (and will continue to reveal) spatial and spectral structure leading to new insights in chromospheric phenomena related to magnetic fields and heating, such as Ellerman Bombs (Libbrecht et al., 2016, henceforth Paper I). iii List of Papers Paper included in the Thesis Paper I Libbrecht, T., Joshi, J., de la Cruz Rodríguez, J., Leenaarts, J., and Asensio Ramos, A. (2016). “Observations of Ellerman bomb emission features in He I D3 and He I 10830 Å ”. In: ArXiv e-prints. arXiv: 1610.01321 [astro-ph.SR]. Comment: accepted for publication in A&A. Contribution to Paper I: TL and JJ observed and reduced the data. TL conducted the full analysis, made all the figures and wrote the entire paper. Papers not included in the Thesis Leenaarts, J., Golding, T., Carlsson, M., Libbrecht, T., and Joshi, J. (2016). “The cause of spatial structure in solar He I 1083 nm multiplet images”. In: ArXiv e-prints. arXiv: 1608.00838 [astro-ph.SR]. Comment: accepted for publication in A&A. Melandri, A., Bernardini, M.G., D’Avanzo, P., Sanchez-Ramirez, R., Nappo, F., Nava, L., Japelj, J., de Ugarte Postigo, A., Oates, S., Campana, S., Covino, S., D’Elia, V., Ghirlanda, G., Gafton, E., Ghis- ellini, G., Gnedin, N., Goldoni, P., Gorosabel, J., Libbrecht, T., Malesani, D., Salvaterra, R., Thone, C.C., Vergani, S.D., Xu, D., and Tagliaferri, G. (2015). “The high-redshift gamma-ray burst GRB140515A. A comprehensive X-ray and optical study.” A&A 581, A86. arXiv: 1506.03079. Non-Refereed Items GCN #16253,#16278,#16290,#16310 v Contents Abstract iii List of Papers v 1 Introduction to the Solar Atmosphere 1 2 Helium Line Formation 5 2.1 Discovery of Helium ............................... 5 2.2 Atomic Structure................................. 6 2.3 Line Formation Mechanisms........................... 7 2.4 Review on Helium Line Formation Research.................. 9 2.4.1 Helium Line Intensity .......................... 9 2.4.2 Coronal Heating and Early Measurements of the Solar UV Flux (50’s-70’s) 10 2.4.3 Observational Constraints........................ 11 2.4.4 Semi-Emperical 1D Model Atmospheres ................ 12 2.4.5 Diffusion and Burst Models for Helium Line Formation . 15 2.4.6 Non-Equilibrium Helium Ionization and 3D Modelling . 16 3 Polarization and Inversion Codes 21 3.1 Polarization of Light ............................... 21 3.2 Polarizing Mechanisms.............................. 22 3.2.1 Zeeman effect............................... 22 3.2.2 Atomic level polarization......................... 24 3.2.3 Hanle effect................................ 25 3.2.4 Regimes.................................. 26 3.3 The Polarized Radiative Transfer Equation................... 27 3.4 Inversion of Observed Stokes Parameters of Helium Lines........... 28 3.4.1 Levenberg-Marquardt algorithm..................... 29 3.4.2 HELIX+ .................................. 30 3.4.3 HAZEL ................................... 32 3.4.4 Ambiguities................................ 34 4 Observations of He i D3 37 4.1 Telescopes and Instruments ........................... 37 4.1.1 Fabry-Pérot Interferometer versus Slit-Spectrograph.......... 37 4.1.2 Overview of Facilities........................... 40 4.2 Spectral Profiles of He I D3 and He I 10830 Å ................. 41 4.3 Overview of He I D3 Observations and Results................. 42 4.3.1 Prominences and Filaments....................... 42 4.3.2 Spicules.................................. 45 vii 4.3.3 Flares ................................... 47 4.3.4 On-disk Targets: Active Regions and Flux Emergence......... 50 5 Ellerman bombs 53 5.1 Definition..................................... 53 5.2 Flux Emergence and Magnetic Reconnection.................. 54 5.3 Spectral Signatures and Modelling ....................... 56 6 Summary and Outlook 59 Bibliography 61 Paper I 77 viii Introduction to the Solar Atmosphere 1 Getting a paper out of a PhD student is like getting „the last dried-up bit of ketchup out of a plastic bottle. — Göran Scharmer ISP Throughout history, the Sun has been studied from the Earth by means of radiation (and particles) originating in the solar atmosphere. The convection zone can be studied observationally using helioseismology, and the only probe for the core of the Sun are solar neutrino’s. Apart from those, the study of radiation escaping from the solar atmosphere is our only way of observing the Sun. The layer where photons in the optical continuum escape is called the photosphere. When observing the Sun in the optical continuum or in photospheric spectral lines (such as most Fe I lines), the granulation pattern becomes evident. This pattern emerges as a result of convective motions, giving rise to bright granules where plasma rises surrounded by darker and narrow intergranular lanes in which the plasma flows down. Magnetic features become apparent in the photosphere in the form of magnetic bright points, pores and sunspots (see e.g. the cover image of this thesis). An important parameter when studying the solar atmosphere is the plasma-β parameter, defined as the ratio between the gas pressure Pgas and the magnetic pressure Pmagn: Pgas 2µ0Pgas β = = 2 , (1.1) Pmagn B with µ0 the permeability of the vacuum and B the magnetic field strength. The photosphere consists of high-β plasma. The density is high meaning that collisions govern line formation in the photosphere and therefore, most photospheric lines can assumed to be formed in local thermodynamical equilibrium (LTE). The chromosphere is the layer situated above the photosphere. In the chromosphere, the pressure drops by several orders of magnitude over a short distance (500-1000 km) which completely changes the physical conditions in the atmosphere. The chromosphere is a low-β plasma in which the magnetic force dominates the dynamics. The chromosphere is usually observed in the core of the strongest lines of the solar spectrum, such as hydrogen Balmer lines, and resonance lines of Ca II and Mg II. These lines have such large opacities that line core photons escape from the chromosphere while line wing photons escape from the photosphere. Chromospheric features are entirely different from photospheric ones and include canopies of fibrils, loops, jets, etc. (see Fig. 1.1). Due to the small density, collisional rates are low so that the approximation of LTE is typically invalid for chromo- spheric lines, which presents a challenge to modelling these lines. Additional complications to chromospheric modelling are: effects of partial and non-equilibrium ionization, partial frequency redistribution of scattered photons, and 3D radiative transfer effects. A review of chromospheric diagnostics and their required modelling efforts is given in Section 4 of de la Cruz Rodríguez and van Noort (2016). 1 Fig. 1.1: The chromosphere as seen in the Hα line core λ0 (left panel). The right panel shows the Hα red wing at λ0 + 1.05Å with contributions from both the chromosphere and upper photosphere. Observed with SST/CRISP by Jaime de la Cruz Rodríguez and Jorrit Leenaarts. The transition region is the interface region between the chromosphere and corona in which the temperature suddenly and very steeply rises from O(104) K to O(106) K. The steep temperature rise occurs when all neutral hydrogen (and helium) is ionized away. Lines of highly ionized species become visible such as Si IV and O IV lines. The transition region is a topic of many recent and present studies, due to the launch of the Interface Region Imaging Spectrograph in 2013 (IRIS, De Pontieu et al., 2014). The hot corona is sampled in lines of highly ionized species (e.g. Fe XII 193 Å or Fe XVIII 94 Å) from space with instruments such as the Atmospheric Imaging Assembly (AIA, Lemen et al., 2012) on board of the Solar Dynamics Observatory. Observable features in the corona are for example large scale magnetic loops above active regions
Details
-
File Typepdf
-
Upload Time-
-
Content LanguagesEnglish
-
Upload UserAnonymous/Not logged-in
-
File Pages101 Page
-
File Size-