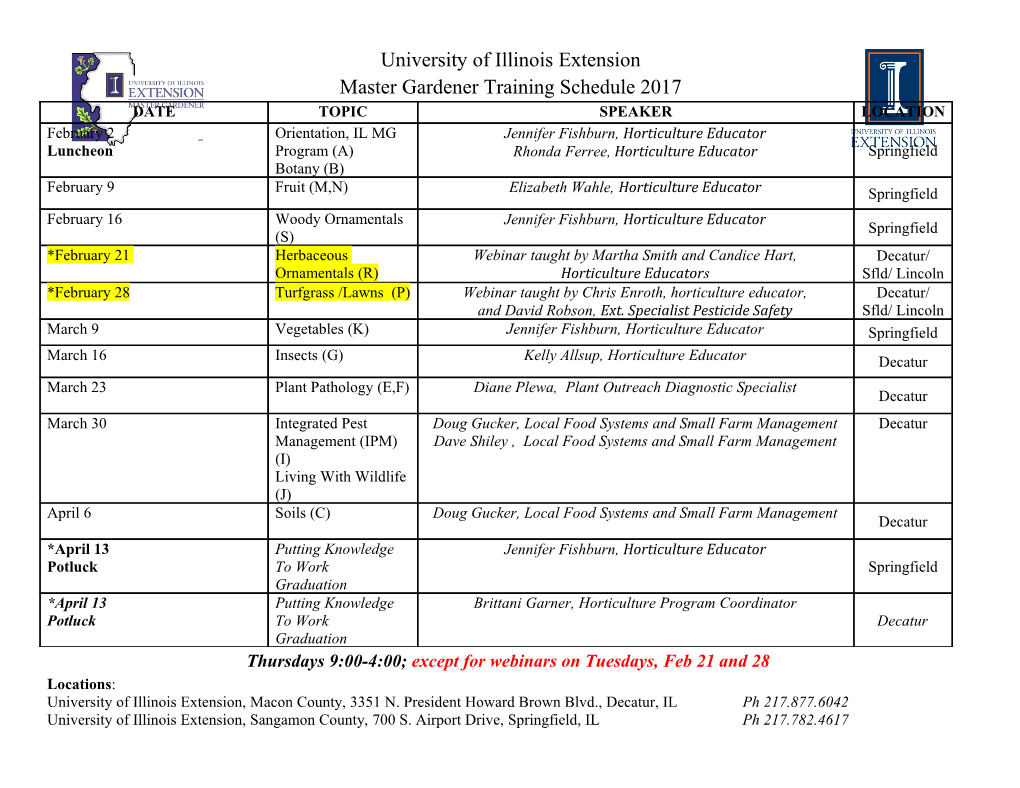
Source: STANDARD HANDBOOK OF BIOMEDICAL ENGINEERING AND DESIGN P•A•R•T•3 BIOMATERIALS Downloaded from Digital Engineering Library @ McGraw-Hill (www.digitalengineeringlibrary.com) Copyright © 2004 The McGraw-Hill Companies. All rights reserved. Any use is subject to the Terms of Use as given at the website. BIOMATERIALS Downloaded from Digital Engineering Library @ McGraw-Hill (www.digitalengineeringlibrary.com) Copyright © 2004 The McGraw-Hill Companies. All rights reserved. Any use is subject to the Terms of Use as given at the website. Source: STANDARD HANDBOOK OF BIOMEDICAL ENGINEERING AND DESIGN CHAPTER 11 BIOPOLYMERS Christopher Batich and Patrick Leamy University of Florida, Gainesville, Florida 11.1INTRODUCTION 11.3 11.2POLYMER SCIENCE 11.4 11.3SPECIFIC POLYMERS 11.12 REFERENCES 11.30 11.1 INTRODUCTION Polymers are large molecules synthesized from smaller molecules called monomers. Most polymers are organic compounds with carbon as the base element. Plastics are polymers that are rigid solids at room temperature and generally contain additional additives. Some common plastics used in bio- medical applications are polymethyl methacrylate for intraocular lenses, braided polyethylene tereph- thalate for vascular grafts, and ultrahigh-molecular-weight polyethylene for the articulating surfaces of orthopedic implants. Polymers and biopolymers in particular encompass a much broader spectrum than plastics alone. Biopolymers include synthetic polymers and natural polymers such as proteins, polysaccharides, and polynucleotides. This chapter will only cover the most commonly used ex- amples in each class but will provide references to more specific sources. Many useful polymers are water-soluble and are used as solutions. Hyaluronic acid is a naturally occurring high-molecular-weight polymer found in connective tissues and is used to protect the iris and cornea during ophthalmic surgery. Polyvinyl pyrrolidinone is a synthetic polymer used as a binder or additive in 25 percent of all Pharmaceuticals.1 Hydrogels are another class of polymers that has many biomedical applications. Hydrogels are polymers that swell in water but retain their overall shape. They are therefore soft and moist and mimic many natural tissues. The most wellknown hydrogel series is poly(hydroxyethyl methacrylate) (PHEMA) and PHEMA copolymers, which are used in soft contact lenses. Gelling polymers are hydrogels that can be formed in situ using chemical or physical bonding of polymers in solution. Alginates, for instance, are acidic polysaccharides that can be cross-linked using divalent cations such as calcium. Other examples of gelling polymers are the poloxamers that can gel with an increase in temperature. Alginates are widely used in cell immobilization, and poloxamers are used as coatings to prevent postsurgical adhesions. Elastomers are low-modulus polymers that can reversibly deform up to many times (some over 500 percent) their original size. Silicones and polyurethanes are common elastomeric biopolymers. Polyurethane is used as a coating for pacemaker leads and for angioplasty balloons. Silicones are used for a variety of catheters, soft contact lenses, and foldable intraocular lenses. 11.3 Downloaded from Digital Engineering Library @ McGraw-Hill (www.digitalengineeringlibrary.com) Copyright © 2004 The McGraw-Hill Companies. All rights reserved. Any use is subject to the Terms of Use as given at the website. BIOPOLYMERS 11.4 BIOMATERIALS The next section begins with an overview of polymer science topics, including synthesis, structure, and mechanical properties. The remainder of this chapter (Sec. 11.3) will discuss individual polymers, including their applications and properties. The polymers are presented in the following order: water-soluble polymers, gelling polymers, hydrogels, elastomers, and finally, rigid polymers. These five categories are roughly ordered from low to high modulus (i.e., high to low compliance). Water-soluble polymers in solution do not have an elastic modulus since they are fluids, so these are presented first. In fact, most polymers do not have a true elastic modulus since they are viscoelastic and exhibit solid and viscous mechanical behavior depending on the polymer structure, strain rate, and temperature. Natural tissues are continuously repaired and remodeled to adjust to changes in the physiologic environment. No current synthetic biomaterial or biopolymer can mimic these properties effectively. Consequently, the ideal biomaterial or biopolymer performs the desired function, eventually disappears, and is replaced by natural tissue. Therefore, degradable polymers are of great interest to the biomedical engineering community. Polylactides and their copolymers are currently used as bone screws and sutures since they have good mechanical properties and degrade by hydrolysis so that they can, under optimum conditions, be replaced by natural tissue. In addition to classification as water-soluble polymers, gelling polymers, hydrogels, elastomers, and rigid polymers, polymers can also be classified as bioinert, bioerodable, and biodegradable. Bioinert polymers are nontoxic in vivo and do not degrade significantly even over many years. Polymers can degrade by simple chemical means or under the action of enzymes. For the purposes of this chapter, bioerodable polymers such as polylactide are those that degrade by simple chemical means, and biodegradable polymers are those that degrade with the help of enzymes. Most natural polymers (proteins, polysaccharides, and polynucleotides) are biodegradable, whereas most synthetic degradable polymers are bioerodable. The most common degradation reactions for bioerodable polymers are hydrolysis and oxidation. 11.2 POLYMER SCIENCE 11.2.1 Polymer Synthesis and Structure Polymers are frequently classified by their synthesis mechanism as either step or chain polymers. Step polymers are formed by step wise reactions between functional groups. Linear polymers are formed when each monomer has two functional groups (functionality = 2). The second type of polymeriza- tion is chain polymerization, where monomers are added one at a time to the growing polymer chain. Most polymerization techniques yield polymers with a distribution of polymer molecular weights. Polymer molecular weight is of great interest since it affects mechanical, solution, and melt properties of the polymer. Figure 11.1 shows a schematic diagram for a polymer molecular weight distribution. Number average molecular weight Mn averages the molecular weight over the number of molecules, whereas weight average molecular weight Mw averages over the weight of each polymer chain. Equations (11.1) and (11.2) defined Mw and Mn. (11.1) (11.2) where Ni is the number of polymer chains with molecular weight Mi. The polymerization mechanism is a useful classification because it indicates the likely low- molecular-weight contaminants present. Chain-growth polymers frequently contain unreacted monomers, whereas step-growth polymers have low-molecular-weight oligomers (short chains) present. Downloaded from Digital Engineering Library @ McGraw-Hill (www.digitalengineeringlibrary.com) Copyright © 2004 The McGraw-Hill Companies. All rights reserved. Any use is subject to the Terms of Use as given at the website. BIOPOLYMERS BIOPOLYMERS 11.5 FIGURE 11.1 Typical polymer molecular weight distribution. These low-molecular-weight species are more mobile or soluble than polymers and hence more likely to have physiologic effects. For instance, the monomer of PMMA causes a lowering of blood pressure and has been associated with life-threatening consequences when present (e.g., in some bone cements). Furthermore, the same polymer can be prepared by both mechanisms, leading to different impurities. For instance, polylactide is usually prepared by a chain-growth mechanism involving ring opening of a cyclic dimer (lactide) rather than the condensation of lactic acid. As with all materials, a polymer’s properties can be predicted and explained by understanding the polymer’s structure on the atomic, microscopic, and macroscopic scales. Polymers can be roughly classified into two different classes, thermoplastic and thermoset. Thermoplastic polymers are made of individual polymer chains that are held together by relatively weak van der Waals and dipole-dipole forces. Thermoplastic polymers can be processed into useful products by melt processing, namely, injection molding and extrusion. They can also be dissolved in solvents and cast to form films and other devices. Although they often degrade or denature before melting, most proteins and polysaccharides can be considered thermoplastics since they are made of individual chains and can be dissolved in solvents. Finally, thermoplastics can be linear or branched. Thermosetting polymers contain cross-links between polymer chains. Cross-links are covalent bonds between chains and can be formed using monomers with functionalities of greater than 2 during synthesis. Some polyurethanes and many silicones are formed using monomers with functionalities greater than 2. Cross-links also can be created after the polymer is formed. An example of this is vulcanization, which was discovered by Charles Goodyear in 1839 to toughen natural rubber. Vulcanization uses sulfur as a cross-linking agent. Thermosets are, in essence, one giant molecule since all the polymer chains are connected through the cross-links. Thermosets cannot be melted after they are formed and cannot be dissolved in solvents. Depending on the cross-link
Details
-
File Typepdf
-
Upload Time-
-
Content LanguagesEnglish
-
Upload UserAnonymous/Not logged-in
-
File Pages30 Page
-
File Size-