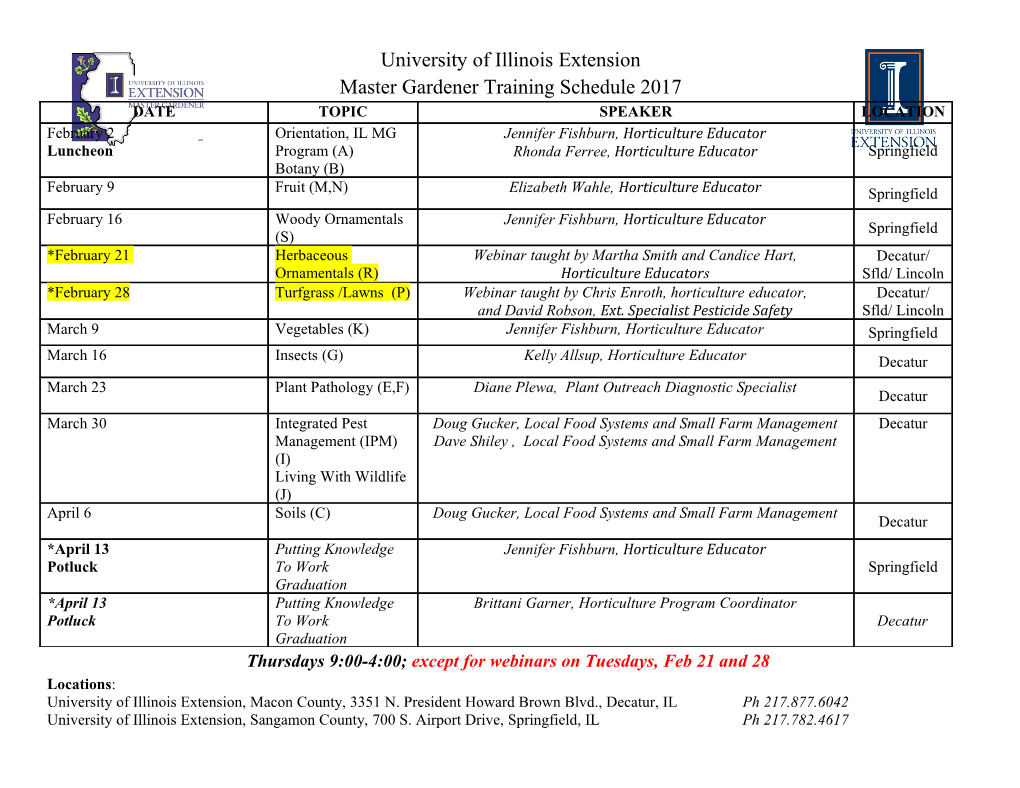
Mastering the interface for advanced all-solid-state lithium rechargeable batteries Yutao Lia,b,1, Weidong Zhoua,b,1, Xi Chena,b,1, Xujie Lüc, Zhiming Cuia,b, Sen Xina,b, Leigang Xuea,b, Quanxi Jiac, and John B. Goodenougha,b,2 aMaterials Science and Engineering Program, The University of Texas at Austin, Austin, TX 78712; bTexas Materials Institute, The University of Texas at Austin, Austin, TX 78712; and cCenter for Integrated Nanotechnologies, Los Alamos National Laboratory, Los Alamos, NM 87545 Contributed by John B. Goodenough, September 30, 2016 (sent for review August 24, 2016; reviewed by Ken Poeppelmeier and Jean-Marie Tarascon) A solid electrolyte with a high Li-ion conductivity and a small The stability of the solid electrolyte on contact with a lithium interfacial resistance against a Li metal anode is a key component anode is a critical issue. If a lithium anode reduces the electro- in all-solid-state Li metal batteries, but there is no ceramic oxide lyte, (i) the electrolyte may become an electronic conductor, + electrolyte available for this application except the thin-film Li-P (ii) an interface layer may form that blocks Li transfer, or (iii)an + oxynitride electrolyte; ceramic electrolytes are either easily re- interface layer may form that conducts Li ions with a low im- duced by Li metal or penetrated by Li dendrites in a short time. pedance. The third situation forms with a LiZr2(PO4)3 electro- + Here, we introduce a solid electrolyte LiZr2(PO4)3 with rhombohe- lyte. In this paper, we report that a Li electrolyte with the dral structure at room temperature that has a bulk Li-ion conduc- NASICON structure, LiZr2(PO4)3, can be fabricated by using −4 −1 tivity σLi = 2 × 10 S·cm at 25 °C, a high electrochemical stability + + zirconium acetate as the precursor and spark plasma sintering up to 5.5 V versus Li /Li, and a small interfacial resistance for Li + + (SPS); it forms a stable Li -conducting SEI that is wet by a transfer. It reacts with a metallic lithium anode to form a Li -con- metallic lithium anode and also wets the electrolyte to provide a ducting passivation layer (solid-electrolyte interphase) containing safe, all-solid-state Li/LiFePO4 cell operating at Top = 80 °C with Li P and Li ZrO that is wet by the lithium anode and also wets the 3 8 6 a long cycle life; the LiFePO4 cathode particles are embedded in LiZr2(PO4)3 electrolyte. An all-solid-state Li/LiFePO4 cell with a polymer a polymer catholyte and carbon. catholyte shows good cyclability and a long cycle life. CHEMISTRY Results and Discussion solid electrolyte | lithium anode | polymer catholyte | interfaces | NASICON The X-ray diffraction (XRD) results of as-prepared LiZr2(PO4)3 are shown in Fig. 1A; the phase of LiZr2(PO4)3 depended on the + rechargeable cell having a flammable organic liquid Li starting materials. Although different zirconium salts decomposed T < B Aelectrolyte has enabled the wireless revolution, but it is not at the same temperature 400 °C (Fig. 1 ), a pure LiZr2(PO4)3 able to power safely an electric road vehicle at a cost that is phase with rhombohedral structure could only be obtained with competitive with the gasoline-powered internal combustion en- zirconium acetate; a triclinic phase with space group C-1was gine (1–4). Safety concerns as well as cost, volumetric energy obtained with other zirconium materials. LiZr2(PO4)3 prepared by density, and cycle life have prevented realization of a commer- solid-state reaction with ZrO2 as the starting material was repor- cially viable electric road vehicle. To address this problem, ted to change from triclinic to rhombohedral structure at 60 °C considerable effort is being given to the development of a solid (13, 14). The rhombohedral LiZr2(PO4)3 phase with high Li-ion + + Li or Na electrolyte that is wet by a metallic lithium or sodium conductivity was stable at room temperature with zirconium ace- −4 −1 anode and has an alkali ion conductivity σi > 10 S·cm at the tate as the starting zirconium salt. The XRD refinement of the C cell operating temperature Top, where a Top ≤ 25 °C is desired rhombohedral LiZr2(PO4)3 phase is shown in Fig. 1 with re- (5–10). Such a development would allow new as well as tradi- liability factors (Rwp = 11.0%; RB = 2.3%). The rhombohedral tional strategies for the cathode. Wetting of the solid electrolyte surface is desired not only because it prevents dendrite forma- Significance tion and growth during plating of an alkali metal anode, but also because wetting constrains the anode volume change in a charge/ Realization of a safe, low-cost rechargeable lithium battery of discharge cycle to be perpendicular to the anode/electrolyte in- high energy density and long cycle life is needed for powering terface, thereby allowing a long cycle life. Therefore, the shear an electric road vehicle and for storing electric power gener- modulus of the electrolyte may not be critical where lithium wets ated by solar or wind energy. This urgent need has prompted the electrolyte surface. efforts to develop a solid electrolyte with an alkali metal an- Ceramic oxide electrolytes offer a large energy gap between ode. Only now is it recognized that the key requirement is their conduction and valence bands, which can allow realization wetting of the electrolyte surface by the alkali-metal anode. of a battery cell with a large energy separation between the an- We report a full rechargeable cell with a solid electrolyte that, ode and cathode chemical potentials without either reduction or although it is reduced by metallic lithium, forms a thin lithium– electrolyte interface that is wet by the anode and wets the oxidation of the electrolyte by an electrode (2). However, if an + alkali-metal anode reduces the solid electrolyte, formation of a electrolyte to give a small Li transfer resistance across the stable solid-electrolyte interphase (SEI) that conducts the working interface. + + + + Li or Na ion is acceptable if the Li or Na transfer across the SEI + Author contributions: Y.L. and J.B.G. designed research; Y.L., W.Z., X.C., Z.C., S.X., and L.X. has a low resistance. Although many ceramic solid Li electrolytes performed research; Y.L., W.Z., X.L., and Q.J. analyzed data; and Y.L. and J.B.G. wrote have been investigated, they are easily reduced by Li metal and/or the paper. they have failed to block dendrite formation and growth into their Reviewers: K.P., Northwestern University; and J.-M.T., Collège de France. grain boundaries (SI Appendix,Fig.S1). However, the rhombo- The authors declare no conflict of interest. hedral structure of the Na electrolyte Na1+3xZr2(SixP1–xO4)3 (11), 1Y.L., W.Z., and X.C. contributed equally to this work. NASICON, which was developed over 45 y ago, has recently been 2To whom correspondence should be addressed. Email: [email protected]. used in a cell design in which a seawater cathode provides the so- This article contains supporting information online at www.pnas.org/lookup/suppl/doi:10. dium of the anode (12). 1073/pnas.1615912113/-/DCSupplemental. www.pnas.org/cgi/doi/10.1073/pnas.1615912113 PNAS Early Edition | 1of5 Downloaded by guest on October 1, 2021 Fig. 1. (A) XRD patterns of LiZr2(PO4)3 prepared with different starting materials. (B) Thermogravimetric analysis (TGA) curves of LiZr2(PO4)3 with different starting materials. (C) Rietveld analysis of the XRD data of rhombohedral LiZr2(PO4)3.(D) SEM image of rhombohedral LiZr2(PO4)3 prepared by SPS at 1,000 °C for 10 min. phase has a space group R-3c with lattice parameters a = 8.8442 Å which is smaller than that (0.40 eV) of LiZr2(PO4)3 prepared by and c = 22.2645 Å, which is very close to those of LiZr2(PO4)3 at solid-state reaction (15, 16). In rhombohedral LiZr2(PO4)3 400 °C prepared by solid-sate reaction. A pure rhombohedral (Fig. 1C, Inset), 90% and 10% Li ions occupy, respectively, the LiZr2(PO4)3 phase was also obtained by SPS. The electron-dis- sixfold disordered 36f M1 sites and the threefold disordered 18e persive spectroscopy mapping in SI Appendix,Fig.S2indicates a M2 sites (13). The interstitial space is large enough for Li-ion uniform distributions of Zr, P, and O elements in the LiZr2(PO4)3 transport, and higher Li-ion conductivity may be obtained by pellet. The LiZr2(PO4)3 pellets fired at 1,150 °C for 20 h in a box doping with different valent ions to increase the Li-ion population furnace and fired at 1,000 °C for 100 min by SPS have a density of inside the framework. –3 –3 + 85% (2.66 g·cm , SI Appendix,Fig.S3) and 99.9% (3.15 g·cm , Whereas a garnet Li electrolyte can have a higher bulk σLi = −3 −1 Fig. 1D), respectively. 1 × 10 S·cm at 25 °C (17), an insulating Li2CO3 surface layer A pure rhombohedral LiZr2(PO4)3 phase can be obtained forms on exposure to moist air; the interfaces of a symmetric Li/ + + + as a well-sintered ceramic at 1,200 °C by Y3 or Ca2 doping for garnet/Li cell create a large resistance to Li transfer between + − Zr4 ; the rhombohedral structure remains unchanged from –70 °C the anode and the electrolyte (about 1,700 Ω·cm 2, SI Appendix, to 200 °C (15). The acetate route supplies particles with the Fig. S5) that dominates the total resistance of the electrolyte.
Details
-
File Typepdf
-
Upload Time-
-
Content LanguagesEnglish
-
Upload UserAnonymous/Not logged-in
-
File Pages5 Page
-
File Size-