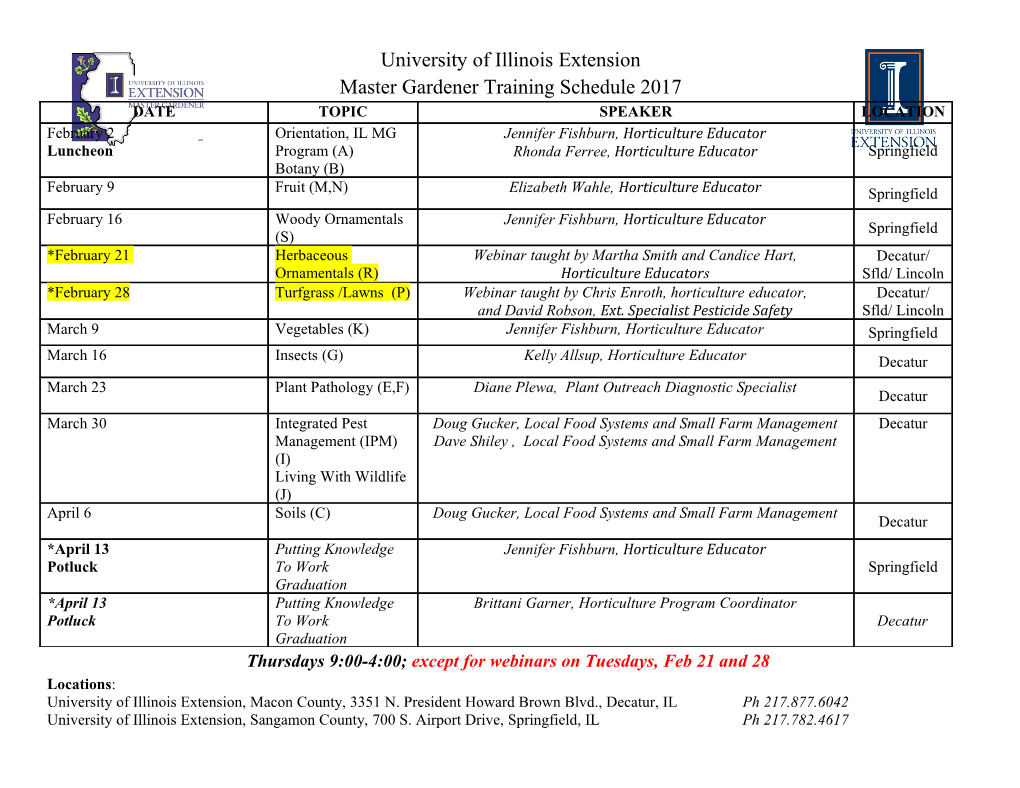
Reciprocating Engine Power Solutions in the Era of Hybrids: Optimizing Integrated Power Plant Design Author: Erlend Vaktskjold, Technology Specialist, Bergen Engines AS, Rolls-Royce Power Systems Co-author and presenter: Leif-Arne Skarbø, Chief Technology Officer, Bergen Engines AS, Rolls-Royce Power Systems Co-author: R.M. Bhaskar, Director - System Project Delivery, Bergen Engines AS, Rolls-Royce Power Systems 1. Introduction Predicting the future is a challenging task, but when it comes to power generation mix, one thing seems certain – the penetration of variable renewable energy sources will continue to grow over time as predicted in many energy outlook reports, most recently DNV-GL’s Energy Transition Outlook 2018. Figure 1. Historical and predicted world electricity generation by power type. Note the remarkable overall growth in electricity energy demand, and the growth in wind and solar PV: only these technologies show a significant increase in 2050 compared to 2016. While many technologies exist for harvesting energy from the atmosphere, from running rivers, from the sea and from ground heat, wind mills and solar cells are dominating the currently seen growth in installed power. In many markets, energy from onshore wind and solar cells is competitive without any form of subsidy. As costs continue to fall, these renewable energy sources will become more competitive, in more and more markets. This development will enable not only reduction in cost of electrical energy, but also a reduction in fossil fuel usage, even in DNV-GL’s forecast of worldwide power demand increasing by 160% within 2050 [1]. Rolls-Royce sees this as both a natural and necessary development for the world’s economy: lower cost of energy with less impact on the natural environment is generally good for business. We recognise the need to drastically reduce the emission of climate gases, with the ultimate goal of zero net emissions. However, between now and some happy future with abundance in renewable energy supply and storage solutions sufficiently large to enable 100% renewable despatch-on-demand power, there will be a power mix consisting of mainly thermal, nuclear, hydro, wind and solar photo-voltaic (PV). In this paper we address the role of flexible thermal power plants in the era of de-carbonization. In particular, we shall discuss medium speed reciprocating power plants, and how these can be effectively erected and provide the necessary support for increased penetration of variable renewable energy sources. Definition of terms AC Alternating current ancillary services stable transmission of electrical energy require a variety of operations and capabilities beyond generation and transmission lines. Ancillary services is used as a collective term to cover these capacity factor ratio of annually average power production from a power plant to its peak installed power CHP Combined heat and power – term used to describe a plant where the waste heat energy is used for heating purposes curtailment term used to describe the un-used (actually not produced) energy which could be produced if there was a simultaneous demand dispatchable term used for power plants capable of controlled dispatch, i.e. deliver power to the grid whenever requested genset Short for generating set, in this context an assembly consisting of reciprocating engine driving a synchronous electrical machine for the purpose of generating electrical power kWe Unit of active power, with emphasis that the measure is electrical power. Used here to distinguish the electrical power output from a genset from the mechanical output from the engine. LTSA Long term service agreement – a business model for providing service PV Shorthand for solar photo-voltaic generation of electricity. Another common terms are solar cells or solar panels PWh petawatt-hour – unit of energy 1015Wh. 10PWh = 10.000TWh = 0.86Mtoe (million tonnes of oil equivalents) RoCoF Rate of change of frequency [Hz/s] – number indicating how rapid grid frequency is changing SC Short circuit VAr Unit for reactive power. Used as shorthand for reactive power VRE Variable Renewable Energy – any form of renewable energy resource which in its nature has a variable power output. Typical examples are solar PV and wind turbines whose power output depend on local and immediate weather conditions 2. Renewable Power Problem Ever deepening penetration of variable renewable energy (VRE) resources in a power grid is presently introducing grid stability problems. While this is well described in literature (see e.g.[2] and [3]), a brief summary is provided here for completeness. 2.1. Power Balance The first part of the problem relates to frequency stability. Frequency is a global variable, common across an entire interconnected grid. Any imbalance in demand and generation power will result in a change of frequency. This follows directly from Newton’s second law, relating the sum of torque Σ푇 applied to a rotating mass with inertia 퐽 and angular velocity 휔̇ ∑ 푇 = 퐽휔̇ (1) Traditional power generation technologies use electrical machines who directly relate the speed of rotation 휔̇ of the machines to the frequency 푓 of the oscillating voltage and current on the AC grid. The same holds for a large number of power consumers. Thus in the above equation, the inertia of all connected machines, both generators and consumers, can be added to represent a total mass inertia 퐽 of machines rotating synchronous with the grid frequency. To see how the grid frequency responds to imbalance in generation and demand, equation 1 is rearranged as follows 휔 1 ∑ 푇 1 푃푔푒푛푒푟푎푡푖표푛 − 푃푑푒푚푎푛푑 = ∫ 푑푡 ≈ ∫ 푑푡 (2) 휔푏푎푠푒 2퐻 푇푏푎푠푒 2퐻 푆푏푎푠푒 2 퐽휔푏푎푠푒 where the inertia constant 퐻 of the grid is defined by 퐻 = and 푆푏푎푠푒 is the sum of the base power 2푆푏푎푠푒 (normally the VA rating) of the connected generator capacity [4]. We can see directly the role of the inertia constant. Any power imbalance need to be corrected to maintain a stable frequency, and the time available to react depends on the inertia constant. Imbalances arise continuously due to changes in demand, and occasional trips in generation or transmission. Demand changes can reasonably well be predicted days and hours in advance, based on past experience. Thus, traditional generation control is based on ahead planning of predicted load, with minor generation capacities reserved for primary control responding immediately to frequency deviation, and secondary control reserves to restore frequency to nominal value and also restore primary control reserve. Tertiary control reserves are then activated to restore the secondary control reserves. Figure 2 show typical time scales for application and withdrawal of generation under primary, secondary and tertiary control. Figure 2. Idealised power-time chart indicating the activation time scales for primary, secondary and tertiary control reserves. Also shown is inertia providing instant resistance to frequency change. Large synchronous areas, with many plants and multiple interconnections, can operate with very stable frequency with as little as 0.5% primary control reserve. This value increase with smaller synchronous area, but also with increased the relative size of the larges plant, or largest transmission segment, in order to sustain an outage of these large units.. Introducing intermittent renewable generation will result in additional imbalance, as error in predicted sun and wind adds to the error in demand prediction. As a result, more primary, and secondary control reserves must be available. While good weather forecasting is necessary to reduce the uncertainty to a minimum, deep VRE penetration requires substantial tertiary control reserves available for those windless, but cloudy, days. 2.2. Inertia Closely related to the power balance is the role of inertia. As shown in equation (2) above, the total mass inertia of machines rotating synchronous with the grid frequency directly determine the rate-of- change-of-frequency (RoCoF) and peak frequency deviation resulting from instantaneous and the time- integral of power imbalance respectively. More rotating mass means a stiffer grid in terms of its frequency. While conventional power generation are tied to the grid through synchronous generators, PV generation has no rotating mass, and most wind turbines are de-coupled from grid frequency through power-electronic interfaces. Thus deep VRE penetration not only increase unpredictable and predictable power imbalance, but also reduce the inertia constant of the grid. For this reason, the concept of virtual inertia may become indispensable. An increasing amount of study and practical implementation of virtual inertia can be found in literature, e.g. [5] 2.3. Voltage stability While frequency is a global variable within a synchronous area, voltage is a local variable –it’s relative value between 3-phases, voltage with respect to earth, and phase angles relative to phase currents. Conventional generators with synchronous machines have the capacity drive reactive loads, and to instantly and automatically generate large currents in case of low voltage event such, as a short circuit: The peak current may rise to 8 times the rated current. These machines can sustain such high currents for several seconds without sustaining any damage owing to the thermal capacity and low electrical resistance of copper windings. High currents flowing into a fault are reliably measured and is used to isolate the fault, typically within a fraction of a second. VRE is typically connected through power
Details
-
File Typepdf
-
Upload Time-
-
Content LanguagesEnglish
-
Upload UserAnonymous/Not logged-in
-
File Pages26 Page
-
File Size-