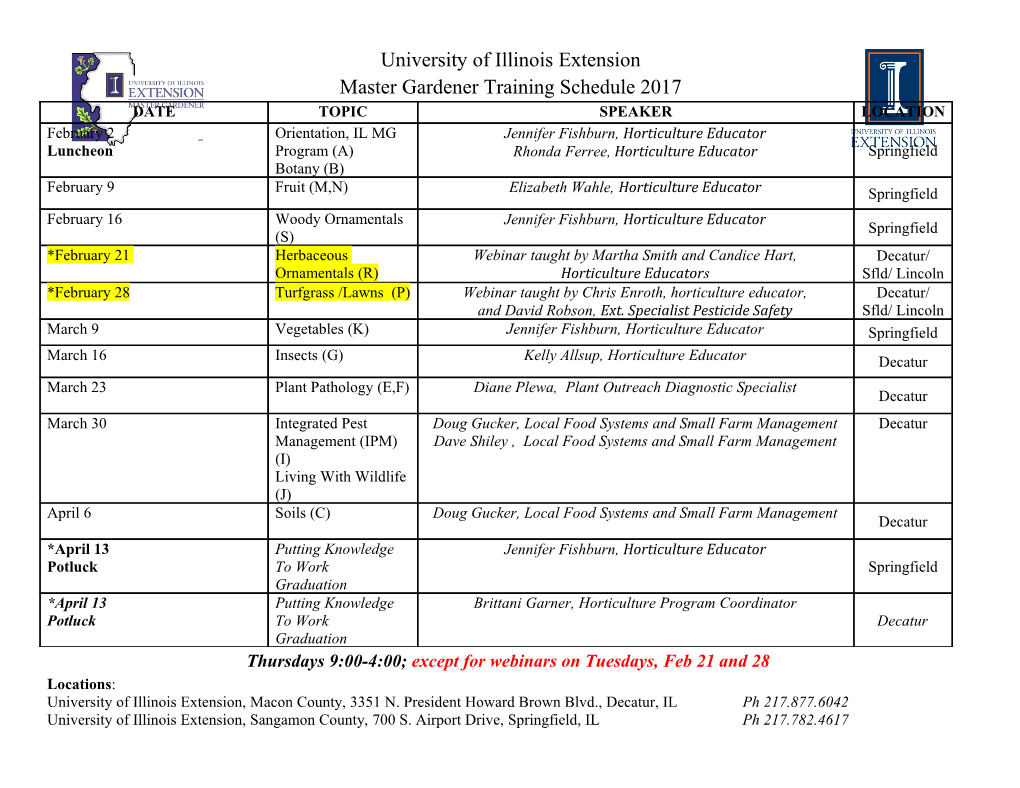
High-speed odor transduction and pulse tracking by insect olfactory receptor neurons Paul Szyszkaa,b,1, Richard C. Gerkina, C. Giovanni Galiziab, and Brian H. Smitha aSchool of Life Sciences, Arizona State University, Tempe, AZ 85287; and bDepartment of Biology, University of Konstanz, 78464 Konstanz, Germany Edited by Lynn M. Riddiford, Howard Hughes Medical Institute, Janelia Farm Research Campus, Ashburn, VA, and approved October 17, 2014 (received for review June 27, 2014) Sensory systems encode both the static quality of a stimulus (e.g., Results color or shape) and its kinetics (e.g., speed and direction). The We constructed an odor delivery device capable of delivering limits with which stimulus kinetics can be resolved are well high frequency pulses (Fig. 1A and Fig. S1). Using titanium understood in vision, audition, and somatosensation. However, tetrachloride (TiCl4) in separate experiments to visualize stimuli, the maximum temporal resolution of olfactory systems has not we estimated that this device delivers odor pulses to the antenna been accurately determined. Here, we probe the limits of temporal in 3.3 ± 0.3 ms (mean ± SD) after triggering the valve to open resolution in insect olfaction by delivering high frequency odor (Fig. 1C). We produced repetitive pulses at frequencies of up pulses and measuring sensory responses in the antennae. We to 200 Hz. To control for responses to mechanical stimuli in show that transduction times and pulse tracking capabilities of experiments with olfactory stimuli, we alternated odor and blank olfactory receptor neurons are faster than previously reported. (odorless) stimuli and subtracted a blank control from the pre- Once an odorant arrives at the boundary layer of the antenna, ceding odor-evoked EAG signal (Fig. S2). odor transduction can occur within less than 2 ms and fluctuating Odor-evoked mean EAG responses began between 1.6 and odor stimuli can be resolved at frequencies more than 100 Hz. 26.4 ms after odors arrived at the antenna (Fig. 1B). EAG re- Thus, insect olfactory receptor neurons can track stimuli of very sponse latency depended on odor identity and decreased with short duration, as occur when their antennae encounter narrow increases in concentration (Fig. 1C). The shortest EAG response filaments in an odor plume. These results provide a new upper onset latencies ranged from 1.6 ms in locusts to 4.6 ms in moths, bound to the kinetics of odor tracking in insect olfactory receptor NEUROSCIENCE and there were no systematic differences between general odors neurons and to the latency of initial transduction events in and species-specific sex or alarm pheromones (Fig. 1C). olfaction. To test antennal pulse tracking capability we applied a 1-s-long series of odor pulses at intervals ranging from 6 to 100 ms olfaction | olfactory receptor neurons | odor transduction | (167–10 Hz; Figs. 2 and 3). Pulse tracking capability decreased temporal resolution | insect with increasing pulse frequency and differed between odor- ants and concentrations, and the maximum pulse tracking dors carried in air plumes quickly break up into thin fila- frequencies ranged from 50 Hz in the orange spotted cock- Oments that spread out across short distances from an odor roach to 125 Hz in the honey bee and locust (Fig. 3 A and B; source (1). The ability to track the temporal structure of filaments see Fig. S3A for variability across antennae). in an odor plume is essential for insects to segregate concurrent Filaments in natural odor plumes arrive at random intervals odors that arise from different sources (2–6). However, it is not and persist for random durations (1). We mimicked this type of clear whether signal transduction times and tracking rates of ol- pattern by applying a 10-s-long broadband frequency stimulus factory receptor neurons (ORNs) are fast enough to allow animals train with random pulse durations and intervals (Fig. 4A). Insect to use the higher frequency components of information present in EAG responses have approximately linear frequency response odor plumes. Insect odor-guided behavior is remarkably robust functions over a wide frequency range, and coherence analysis against the spatial and temporal variability inherent in olfactory stimuli. For example, moths and beetles use temporal stimulus Significance cues to segregate concurrent odors from closely spaced sources – (2 5), and honey bees can detect 6-ms asynchrony in the onset of How fast can animals smell? Whereas we know how fast our concurrent odor stimuli and use this onset asynchrony to segregate eyes are (in the cinema, images at 24 Hz fuse for humans, concurrent odors (6, 7). These observations of fast temporal res- whereas our retina can resolve flickers at more than 100 Hz), olution challenge the frequent notion that olfaction has slow in- olfactory perception is believed to be slow. After all, we take tegration times relative to other senses. Olfactory transduction a sniff and later another one. Odor plumes in the air, however, speed has never been measured directly, and estimates range from can fluctuate at a millisecond time scale. Here, we show that 10 to 30 ms (8–11). Previous studies suggest that the maximum insect olfactory receptor neurons can have response latencies pulse tracking frequency of ORNs is species specific and ranges shorter than 2 ms and resolve odorant fluctuations at more than from 5 to 50 Hz (12–19). However, these numbers do not match 100 Hz. This high temporal resolution could facilitate odor- the high temporal resolution observed in behavioral studies (2–7). background segregation, and it has important implications for We tested the limits of olfactory transduction speed and pulse underlying cellular processes (transduction), ecology (odor rec- tracking in five different insect species by measuring ORN pop- ognition), and technology (development of fast sensors). ulation responses using electroantennogram (EAG) recordings. Author contributions: P.S., R.C.G., and B.H.S. designed research; P.S. performed research; The amplitude and dynamics of EAG signals are proportional to P.S., R.C.G., C.G.G., and B.H.S. analyzed data; and P.S., R.C.G., C.G.G., and B.H.S. wrote the number of sensilla stimulated (20). They are also affected by the paper. receptor current amplitude, positions of the neurons relative to the The authors declare no conflict of interest. recording electrode, and electrical properties of the antenna itself This article is a PNAS Direct Submission. (21). With appropriate odorless controls for electrical and me- 1To whom correspondence should be addressed. Email: [email protected]. chanical artifacts, measuring EAG signals provides reliable esti- This article contains supporting information online at www.pnas.org/lookup/suppl/doi:10. mates of transduction latencies and pulse tracking ability of ORNs. 1073/pnas.1412051111/-/DCSupplemental. www.pnas.org/cgi/doi/10.1073/pnas.1412051111 PNAS Early Edition | 1of6 Downloaded by guest on October 1, 2021 A 3 ms pulses, 20 Hz, N=10 3 ms pulses, 167 Hz, N=10 B 10 20 33 50 67 83 Smoke 100 Stimulus (Hz) 125 167 con Min. log power (V²/Hz) Max. 67 83 33 50 20 10 125 167 0 50 100 150 200 0 50 100 150 200 100 Time after first trigger (ms) Frequency (Hz) C 1.0 Bee, N=38 D 0.8 0.6 70 0.4 0.2 60 0.0 Fig. 1. Olfactory transduction onset can occur in −0.2 3.3 5.8 less than 2 ms. (A) Stimulus dynamics visualized 1.0 Moth, N=15 50 with TiCl4 smokeduring20Hzand167Hzpulse 0.8 series. A laser was positioned at the location of the 0.6 antenna, and the resulting light reflectance of the 0.4 40 smoke was recorded with a photodiode (mean ± 0.2 SEM). SE (SEM) is visualized as a shaded area. (B) 0.0 Color-coded periodograms of visualized TiCl4 smoke 30 −0.2 3.3 7.9 26.4 signals show that the odor delivery device is capable 25.2 1.0 Locust, N=12 of producing pulse frequencies between 10 and 167 21.3 0.8 Hz. (C)TiCl4 smoke signals (red, n = 16) and EAG 20 16.0 ± 0.6 15.6 responses to 2-heptanone (black) (mean SEM). For EAG response onset (ms after odor arrival) 0.4 EAG recordings, odor and blank stimuli were alter- 6.3 10.3 5.5 9.9 5.2 5.4 4.4 6.0 4.6 nated, and the blank control was subtracted from 7.9 3.0 10 5.0 0.2 4.2 3.6 3.2 2.8 3.0 2.0 1.9 3.0 every preceding odor-evoked EAG signal. Signals 2.5 2.5 2.7 0.0 1.6 1.8 were averaged across 10 recordings for each antenna. −0.2 3.3 5.1 Smoke 0 n, number of averaged antennae. The values at the 1.0 Hissing c., N=4 vertical lines are the computed onset times in milli- 0.8 blank)), blank)), seconds. (D) Summary of mean EAG response onsets − Iso (6,0) Lin (9,0) Lin (4,0) Lin (2,0) Hex (4,0) Non (9,0) 0.6 Lin (17,0) Lem (3,0) Hex (35,0) Hex (16,0) Hex (11,0) Non (13,1) Lem (11,0) Lem (12,0) Bom (15,0) for different insect species and odors. The TiCl smoke Hept 1 (4,0) Hept 1 (2,0) 4 Hex 0.1 (3,0) 0.4 Hept 1 (38,0) Hept 1 (16,0) Hept 1 (12,0) Hept 0.1 (4,0) Hept 0.1 (33,0) Hept 0.1 (14,0) Hept 0.1 (12,0) Hept 0.01 (4,0) signal onset time (3.3 ms) was subtracted from the Hept 0.01 (30,0) Hept 0.01 (17,0) Hept 0.01 (11,0) 0.2 Hept 0.001 (6,0) EAG onset times to get the real EAG onset.
Details
-
File Typepdf
-
Upload Time-
-
Content LanguagesEnglish
-
Upload UserAnonymous/Not logged-in
-
File Pages6 Page
-
File Size-